The chemistry to mimic ones of nature’s greatest feats still has some hurdles to overcome
In just one hour, more energy reaches the Earth from the sun than is used by humanity in a whole year. Over billions of years, plants have evolved effective ways to harness this abundant resource – absorbing light energy and storing it as sugars, a chemical energy supply that can be drawn upon as needed.
But human societies rely on energy derived from fossil fuels – finite resources that have appalling consequences in terms of climate and global geopolitics. Couldn’t we instead mimic photosynthesis, and convert light into green fuel?
Nature can’t use rare materials, so [plants] use really abundant materials like manganese and they’re able to catalyse this reaction really efficiently
Jenny Zhang, University of Cambridge
For Allen Bard and Marye Anne Fox, this was chemistry’s holy grail – to use solar energy to produce a clean, storable fuel. They wanted to find ways to use light to break the bonds in water to generate hydrogen.
‘There were a number of people working in this field … and so we tried to take that collective wisdom and put together what it would take to be called successful at this stage, and what we thought the possibilities or probabilities were to be successful,’ recalls Bard. ‘So, for example, we said the efficiency – conversion of solar energy into chemical energy – should be 10%. That was just off the top of our head, but it’s been used ever since by a lot of people.’ Beyond this, Bard and Fox proposed that the materials used in a water-splitting device should be stable for at least 10 years.
The green light
In 1972, Kenichi Honda and his student Akira Fujishima reported experiments carried out at the University of Tokyo in Japan, in which light was shone onto a titanium dioxide electrode immersed in water. Electrons excited by the light left behind positive ‘holes’ that could then draw further electrons from the surrounding water molecules. Honda and Fujishima showed that this catalysed oxygen production at the photoanode, while hydrogen was formed at the platinum cathode. It was the first time that water had been split using a light-induced cell.
In the years since, major research efforts in the US, China, Europe and Japan have helped push up efficiencies. This has gradually brought us closer to a viable artificial photosynthesis process – but there’s still a long way to go if these systems are to operate at a cost or scale akin to the fossil fuel industry.
Three years after Bard and Fox’s article, John Turner and Oscar Khaselev at the National Renewable Energy Laboratory in Colorado, US, developed the first ‘artificial leaf’ – an integrated photovoltaic device that could split water using light as the only energy input. This achieved an impressive 12.4% hydrogen production efficiency, but it relied on expensive materials – the key components were a gallium indium phosphide semiconductor and a platinum electrocatalyst.
A decade later, a group led by Daniel Nocera at the Massachusetts Institute of Technology in the US reported a water-splitting system using electrodes made from cheaper, more abundant materials – cobalt, nickel, molybdenum and zinc. In 2011, Nocera’s team produced its own wireless, solar water-splitting device, featuring these low-cost electrodes coated onto a silicon-based semiconductor. Nocera, working with Sun Catalytix, a company he founded to scale-up the system, planned to commercialise the technology. However, he soon abandoned the idea, realising that the technique was still unlikely to compete with cheaper hydrogen produced by steam reforming of fossil fuels.
Stability issues
Another issue that has beset solar water-splitting systems is their stability. Many of the materials used in these devices corrode when brought into contact with water, especially under the extreme pH generated when they operate, something chemists are still seeking to remedy.
‘There’s a two-pronged approach: one is to discover new semiconductor materials, and the other approach is to try to use a protective coating or stabilisation scheme to essentially bring back all these otherwise unstable semiconductor materials,’ explains Yale University’s Shu Hu, who previously worked at the Joint Center for Artificial Photosynthesis (JCAP), the US Department of Energy’s solar fuel hub. ‘It’s hard to say which one will win out eventually, but the recent big efforts on solar water splitting by JCAP and many other research groups have done both, and so far the protective coating scheme has won out.’
The current efficiency record is held by a system created by a team led by Thomas Hannappel from Ilmenau University of Technology, Germany, and California Institute of Technology’s Harry Atwater, who currently serves as JCAP’s director. Their device incorporated a crystalline titanium dioxide layer that protects the photocathode against corrosion while also minimising light absorption and reflection losses. The device achieved 19.3% solar to hydrogen efficiency, approaching the maximum theoretical limit for this design.
While this particular device was only stable for around 20 hours, oxide coatings have been used elsewhere to keep photoelectrodes stable for over a thousand hours, although Hu notes that improvements are still needed. ‘To make it really industrially relevant you need thousands, hundreds of thousands of hours,’ he says. ‘And that’s neither practical, nor achievable, I think, so far. So that’s the challenge.’
Breaking down the problem
Another obstacle is the complexity involved in making large panel-based devices. An alternative, slightly less mature, technology that might be scaled up more easily is based on particulate photocatalysts. These nanosized particles facilitate light absorption, charge separation and catalysis all in one place.
‘Given that you can make both reductive and oxidative reactions happen only a few nanometres apart, that gives you a lot of freedom to design many other different kinds of photochemistries – not just water splitting, but for example, maybe carbon dioxide reduction, or even partial oxidation of alkanes to make fine chemicals,’ says Hu. ‘And then furthermore, if you talk about particles to chemical engineers, they understand immediately, because all these catalytic reactors – crackers and petroleum refineries – they use particles all the time. So the chemical industry knows how to handle those, but knows less about how to handle photoelectrodes.’
Kazunari Domen’s group at the University of Tokyo in Japan is a leading player in particulate photocatalysis and has been investigating photocatalytic water splitting using strontium titanate particles for more than four decades. ‘[In 1980], the water splitting activity of strontium titanate was too low to investigate the quantum yield,’ says Domen.
One problem was stopping electron–hole pairs recombining before they react with water. Domen explains that careful adjustments to the way the photocatalyst and co-catalysts were prepared meant that, by 2018, the apparent quantum yield in overall water splitting had improved to 69% – meaning that 69% of photons illuminating the catalyst could be harnessed for hydrogen production.
Earlier this year, Domen’s group reported a strontium titanate photocatalyst that could convert light energy with almost perfect quantum efficiency. Domen explains that this was made possible by combining techniques developed by various groups over many years. ‘Applying aluminium doping suppresses defect formation and particle growth, flux treatment enhances the crystallinity and exposes {100} and {110} facets of strontium titanate,’ says Domen. With these distinct crystal facets exposed, the team could selectively deposit hydrogen and oxygen evolution co-catalysts on different sides of the strontium titanate particles. The selective migration of electrons and holes to these sites is key to eliminating recombination.
However, Domen explains that there is still much work to be done to improve the overall solar-to-hydrogen efficiency. The problem is that the strontium titanate photocatalyst only makes use of the ultraviolet portion of sunlight, which accounts for around 5% of the total solar energy available. Despite the impressive engineering, when irradiated under simulated sunlight it only has an overall efficiency of 0.65%. In order to produce an economically viable water-splitting system, photocatalysts need to use other parts of the spectrum.
‘It is essential to develop photocatalysts showing similarly high apparent quantum yields in the visible light region,’ says Domen. ‘We are developing some promising visible-light-responsive photocatalytic materials, including oxynitrides, nitrides and oxychalcogenides, which have the potential to split water under visible light. The photocatalyst design revealed from the success of the strontium titanate photocatalyst will advance the development of such visible-light-active photocatalysts.’
Back to nature
In recent years, some researchers have turned to nature for more than just inspiration, actively incorporating biological systems into their water-splitting technologies. In 2012, Nocera teamed up with Harvard Medical School’s Pamela Silver to work on a ‘bionic leaf’. In 2016, they demonstrated a biosynthetic system in which Nocera’s water-splitting catalysts produce hydrogen that then feeds genetically engineered bacteria. The bacteria combine this with carbon dioxide to create liquid fuels like butanol that have advantages over hydrogen in that they are safer to store, and are compatible with existing infrastructure.
Others are developing photoelectrochemical cells using the enzyme responsible for water splitting in nature. ‘At the moment we can make okay catalysts, but they usually require really expensive materials,’ says University of Cambridge electrochemist Jenny Zhang. ‘Whereas when you look at plants, all of them are filled with photosystem II. Nature can’t use rare materials, so [plants] use really abundant materials like manganese and they’re able to catalyse this reaction really efficiently.’
Researchers like Zhang have devised ingenious ways to deposit the photosystem II onto electrodes to create ‘semi-artificial’ water-splitting devices. The record solar-to-hydrogen efficiency for these systems is currently 5.4%, although Zhang notes that this required highly optimised conditions.
Despite all of these advances, the leading technology today is still based on traditional electrolysers. Around 4% of the world’s hydrogen is already produced by electrolysis, so powering this with cheap solar electricity seems an attractive option. State-of-the-art photovoltaic–electrolysis set ups today can achieve over 30% solar-to-hydrogen conversion. However, there are still issues with these systems that mean they are unlikely to produce hydrogen at a price and scale that’s competitive with the fossil fuel industry.
Bard reflects that things haven’t moved as quickly for solar-to-hydrogen technologies as he’d anticipated back in the 1990s, and notes the drawbacks of using hydrogen as a fuel. But he still believes that sunlight holds the key to our future energy needs. ‘Ultimately, it’s going to be solar energy or it’s going to be a really different world. We can’t use coal because it gives these really bad side effects and fairly soon we’ll run out of other fuels,’ he says. ‘If I was making a prediction now, it would be simply solar energy to electricity and making it more and more efficient.’
The author would like to thank Amanda Morris, Thomas Mallouk and Ana Moore for helpful conversations.
Top 20 papers race caption: Allan Bard’s original 1995 Accounts of Chemical Research holy grail article appears on the list at number nine, with 1930 citations. There are several other reviews in the top 20, with the top two positions – and a whopping more than 10,000 citations between them – going to reviews by Nathan Lewis and colleagues, emphasising his important position in the field. It’s interesting to see ‘benchmarking’ papers (at positions three and 14, both from the groups of Thomas Jaramillo with Charles McCrory as first author) that set standards for water splitting electrocatalysts – device performance is an important metric in this area and standardising performance measures to enable comparisons between devices is vital.
Citation network caption: As you would expect, many of the researchers revealed in the co-authorship network analysis also appear in the network of citations (which looks at how researchers in this area cite other researchers). But it also shows some others. For example, Michael Grätzel of EPFL in Switzerland is a very large node in this network, but smaller in the co-authorship one. Jiaguo Yu of Wuhan University of Technology is another example of this.
Co-authorship network caption: This is a big area of research with many separate groups working on somewhat different aspects of the main goal. Nathan Lewis is an important and well established researcher, reflected by the size and colour of his node with many connections. You can also see Kazunari Domen, who has worked in the field for decades, as an imporant and well-connected node. Xinchen Wang, who did his PhD with Domen, is a lighter coloured node (meaning his work has appeared more recently), but already of considerable size.
Geographic timelapse caption: This area shows a much greater geographic spread than some of the other grail areas, with highly cited papers appearing on the map in Saudi Arabia, Iran, Israel and Egypt. It is also notable that there is a big increase in the number of papers from around 2008 – does this represent an increasing interest in sustainable energy and fuels around that time, or key papers stimulating more research?
Additional information
For an explanation of how these data visualisations were built, read Behind the data
Further reading
1 H Wang et al, Low-dimensional semiconductors in artificial photosynthesis: an outlook for the interactions between particles/quasiparticles, ACS Cent. Sci., 2020, 6, 1058 (DOI: 10.1021/acscentsci.0c00540)
2 B Pattengale et al, A conductive metal–organic framework photoanode, Chem. Sci., 2020, 11, 959 (DOI: 10.1039/d0sc04302h)
3 S Assavachin et al, Ferroelectric surface photovoltage enhancement in chromium-doped SrTiO3 nanocrystal photocatalysts for hydrogen evolution, Mater. Adv., 2020, 1, 1382 (DOI: 10.1039/d0ma00463d)
4 Y Xu et al, Nitrogen-doped RuS2 nanoparticles containing in situ reduced Ru as an efficient electrocatalyst for hydrogen evolution, RSC Adv., 2020, 10, 17862 (DOI: 10.1039/d0ra02530e)
5 S Tokarev et al, Electron injection effect in In2 O3 and SnO2 nanocrystals modified by ruthenium heteroleptic complexes, Phys. Chem. Chem. Phys., 2020, 22, 8146 (DOI: 10.1039/c9cp07016h)
6 X Chen et al, Electrical decoupling of microbial electrochemical reactions enables spontaneous H2 evolution, Energy Environ. Sci., 2020, 13, 495 (DOI: 10.1039/c9ee02571e)
7 R Irani et al, The role of ultra-thin MnOx co-catalysts on the photoelectrochemical properties of BiVO4 photoanodes, J. Mater. Chem. A, 2020, 8, 5508 (DOI: 10.1039/d0ta00939c)
8 S Tandon et al, A cubane-type manganese complex with H2 O oxidation capabilities, Sustainable Energy Fuels, 2020, 4, 4464 (DOI: 10.1039/d0se00701c)
9 C Lawley et al, Examining the surface evolution of LaTiOx Ny an oxynitride solar water splitting photocatalyst, Nat. Commun., 2020, 11, 1728 (DOI: 10.1038/s41467-020-15519-y)
10 L Zhou et al, Successes and opportunities for discovery of metal oxide photoanodes for solar fuels generators, ACS Energy Lett., 2020, 5, 1413 (DOI: 10.1021/acsenergylett.0c00067)
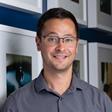
Searching for the holy grails of chemistry
- 1
- 2
- 3
- 4
- 5
- 6
- 7
Currently reading
Has the artificial leaf’s time come at last?
- 8
- 9
No comments yet