Researchers are struggling to agree on the underlying reasons for accelerated rates and altered reaction mechanisms in water microdroplets. Here’s what we do know and where open questions lie…
What are water microdroplets?
They’re just what they sound like: really tiny water droplets. A microdroplet is around 10μm in size, which is about 100 times smaller than a raindrop..
Water microdroplets are the focus of a live and contentious scientific debate. Studies suggest they can accelerate the rates of numerous organic reactions – in some cases by as much as 10,000 times – as well as initiate reactions that do not occur in bulk water solutions. The implications are wide-ranging and extend from explaining prebiotic chemistry to enhancing synthetic chemistry. If chemists can understand them better, and work out how to scale reactions that use them, water microdroplets could help researchers avoid expensive catalysts or high temperatures and pressures when making numerous chemicals.
How do you generate water microdroplets?
There are various ways including using a high-pressure gas nebuliser or a commercial ultrasonic humidifier, which turn bulk water into a spray. An alternative method is letting water vapour condense on a very cold substrate. In nature, crashing ocean waves and atmospheric processes also generate water microdroplets.
Give me some examples of reactions that microdroplets might modify…
Here’s a list of just a few reports documenting reactions that water microdroplets appear to initiate or accelerate. Some of them combine microdroplets with a traditional catalyst but many are described as catalyst-free. Richard Zare of Standford University in the US, and colleagues, are behind most of the studies but it’s not the only team investigating this intriguing phenomenon.
- Hydrogen peroxide generation
- Methane oxidation
- Hexadecane degradation
- Benzoic acid oxidation to phenol
- Decarboxylative amination of carboxylic acids
- Menshutkin reactions
- Viologen degradation
- Oxidative C–H/N–H cross-coupling
- Aryl carbocations from phenols
- Convert carbon dioxide and nitrogen into urea
- Turn nitrogen into ammonia
It’s early days but these last two examples are probably the most exciting. They suggest that water microdroplets might provide a way to synthesise ammonia at ambient temperatures and pressures, which could help researchers develop a sustainable alternative to the Haber–Bosch process.
How are microdroplets different to bulk water?
Apart from the obvious size difference, what distinguishes microdroplets from the bulk is that they have an interface. Bulk water is broadly considered to serve as an inert environment for many chemical systems. In microdroplets, however, the proportion of molecules at the droplet surface is much larger compared to bulk water in a macroscopic vessel, which alters water’s physicochemical properties.
So how do water microdroplets accelerate chemical reactions?
That’s the million-dollar question; there are several conflicting theories, experimental results and computational simulations surrounding the mechanisms by which water microdroplets accelerate reactions.
Evaporation, which increases reagent concentration, and confinement could explain why reaction rates in bimolecular reactions are enhanced by water microdroplets, but the same reasoning wouldn’t apply to unimolecular reactions. Many therefore attribute the accelerated reaction rates to effects on the microdroplet surface including partial solvation, reagent orientation, extremes of surface acidity or basicity and large electric fields. It could be a combination of several factors.
Electric fields are known to alter reaction rates and selectivity, and sometimes even reaction mechanisms. One theory is that electric double layers, the alignment of free O–H bonds in interfacial water molecules or partial charge transfer creates an electric field at the air–water interface. This electric field is thought to be strong enough to oxidise electrons out of hydroxide ions or other closed-shell molecules dissolved in water, forming highly reactive radicals and free electrons.
Are there any other theories?
Yes. One of the most eye-catching reports of microdroplet phenomena is that they can spontaneously form hydrogen peroxide. However, that claim is disputed and a recent preprint attributes this particular effect to a different interface – that between water microdroplets and a solid. Here, the solid is thought to oxidise oxygen dissolved in the water to form hydrogen peroxide. The preprint reports that the tendency of solid substrates towards forming hydrogen peroxide relates to its electrochemical potential, with magnesium generating more hydrogen peroxide than silicon wafers.
Why is it so hard to figure out what’s going on?
It’s hard to design control experiments for multi-phase processes that isolate the exact mechanism responsible for accelerated reaction rates in water microdroplets. Droplet size, temperature and concentration are difficult to hold constant. Quantifying reaction species at low concentrations is also challenging and the systems are also sensitive to contamination. Moreover, simulations that faithfully capture important aspects of the air–water interface are computationally demanding.
How practical would it be to scale-up a reaction using microdroplets?
Scaling up reactions in water microdroplets could be challenging, if not economically uncompetitive with conventional large-scale processes, especially regarding the electrical energy required to create microdroplet mists. What’s more, they’d probably require incredibly large volumes of solvent. A system that recycles and resprays the solvent will likely be part of any large-scale reactor that uses water microdroplets.
Did you say water microdroplets could have played a role in the origin of life?
Many experts agree that processes at the air–water interface helped build chemical complexity. For example, research shows that abiotic amino acids readily form amide bonds at the air–water interface of water microdroplets. Given this scenario mimicked conditions thought to have occurred on early Earth, it suggests that atmospheric aerosols or sea spray might have helped initiate the formation of the first biopolymers.
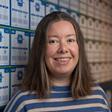
Water microdroplet chemistry is contentious, here’s why
- 1
- 2
Currently reading
Explainer: What are water microdroplets and why are chemists talking about them?
No comments yet