A new catalytic system has been developed that can unravel plastic polymer chains into small chemical fragments. These chemicals are usually obtained by industry from crude oil and this depolymerisation technology can turn used plastics into propylene to make new plastics.
Polyethylene is cheap and strong making it ideal for packaging and single use products – this also means it is the largest contributor to global plastic waste. Current recycling practices generally depend on mechanical processes which degrade the quality of the plastic and therefore limit the use of the recycled material. The inert hydrocarbon chains make these materials particularly resistant to chemical degradation and, although modification can produce upcycled plastics with new properties, the demand for these products is too low to make this a viable recycling strategy. In addition, most plastics are produced from petrochemicals extracted from crude oil, meaning mechanisms to recover these chemical feedstocks are highly desirable.
Chemical recycling is a challenging process, but social and political pressure have increased investment in this area and the field has expanded rapidly over the last 10 years. ‘We’re starting to see a real focus of people’s minds, energy and money on coming up with creative ways to turn our polyolefin waste into something more useful,’ says Andrew Dove, a sustainable polymer chemist at the University of Birmingham, UK.
Researchers in the US have now pioneered a method to depolymerise polyethylene chains into propylene, an important feedstock for the chemical industry. Unusually, two separate teams of scientists simultaneously developed the multi-component catalytic system required for this process: dehydrogenation, followed by a metathesis/isomerisation sequence. The dehydrogenation introduces double bonds into the saturated polymer chain, providing a reactive site for subsequent steps. In the second reaction, ethylene is added across this new double bond, triggering a rearrangement of the bonds along the polymer chain and resulting in the loss of propylene from the end. ‘These are very long molecules, and the bonds that hold them together are very strong,’ explains Susannah Scott, a sustainable catalysis researcher at the University of California, US. ‘Breaking them takes a huge amount of energy, so instead we swap one bond for another to cut the polymer into small pieces without a huge input of energy.’
Scott, and sustainable polymer researcher Damien Guironnet at the University of Illinois Urbana-Champaign, US, were keen to focus on how this chemistry could be used by industry and teamed up with the Dow Chemical Company to develop a scalable reaction system. ‘We wanted to use catalysts that are most relevant for industry,’ says Guironnet. ‘But we also needed to think about the process itself and the type of reactor.’ Having identified a compatible combination of catalysts for the complete reaction sequence, the team designed a semi-continuous flow reactor, allowing separation of the gaseous products from the reaction mixture as they formed. ‘If you can make a reactor do both the reaction and the separation, you save a lot of time, energy and money,’ says Scott. ‘It’s about considering the whole system and finding ways to achieve chemical recycling cheaply and efficiently.’ Scott and Guironnet also conducted a life cycle assessment to evaluate the carbon footprint of the depolymerisation process and reported a 13% reduction in greenhouse gas emissions compared with extracting propylene from crude oil.1
John Hartwig, an organic chemist at the University of California Berkeley, US, took a more fundamental approach, focusing instead on the scope and underlying chemistry of each step in the process.2 ‘One of the biggest problems is that polyethylenes are not all the same. They have different molecular weights, different degrees of branching and they all contain additives,’ he explains. ‘We wanted to make sure that our methodology worked for real post-consumer waste possessing these varying properties.’ The team examined each step of the reaction separately, using isotope labelling to confirm the proposed mechanism and investigating the viability of different polyethylene sources. The catalytic system proved remarkably robust, efficiently depolymerising a range of consumer waste plastics, including shampoo bottles and vegetable packaging. ‘We were surprised to find how tolerant the catalysts were of the impurities added into commercial products,’ Hartwig points out.
Both teams readily acknowledge that this work is decades from becoming a commercial reality but are confident that this method could provide a real solution to a global problem. ‘It’s a fantastic step forward in the field,’ Dove says. ‘Together, both groups have answered the big questions: is it likely to be sustainable? And is it likely to work on real post-consumer waste? The next step is to look at scaling it up and what the real energy costs are going to be.’
The teams will now be focusing on improving the performance and longevity of their catalytic systems with the ultimate aim of expanding this chemistry to other types of plastic. However, Scott is also keen to emphasise the crucial role to be played by chemists in other fields. ‘The world needs to get used to designing plastics for both performance and recycling,’ she says, ‘and that’s going to require new polymer chemistry too.’
References
1 NM Wang et al, J. Am. Chem. Soc., 2022, 144, 18526 (DOI: 10.1021/jacs.2c07781)
2 RJ Conk et al, Science, 2022, 377, 1561 (DOI: 10.1126/science.add1088)
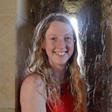
No comments yet