Chiral asparagine monohydrate crystals can segregate by handedness – if you arrange them carefully first
Louis Pasteur’s 1848 experiment that established the hypothesis of molecular chirality is rightly regarded not just as a landmark in structural chemistry but as a paradigm of patience. To separate the two enantiomers of sodium ammonium tartrate, which spontaneously segregated during crystallisation to form mirror-image asymmetric crystals, Pasteur risked eye-strain as he apportioned the little chiral crystals into two piles using tweezers. (The word ‘chirality’, from the Greek for ‘hand’, came decades later, coined by Lord Kelvin in 1893.)
Observing that solutions prepared from each pile rotated the plane of polarised light in opposite directions, Pasteur proposed that this left–right distinction in the interaction of the molecules with light arose from ‘a grouping of the atoms of an asymmetric type that is not superimposable on its mirror image’ – perhaps, he added, because they are ‘situated at the vertices of an irregular tetrahedron’.
It now seems that Pasteur could have employed a less labour-intensive method of separating such chiral crystals – well, in a manner of speaking anyway. A team in China led by Xinhau Wan of Peking University has reported that crystals of asparagine monohydrate about a millimetre long, which also have chiral shapes due to spontaneous segregation of a racemic mixture of both enantiomers of the amino acid, will jump in opposite directions when gently warmed, depending on their handedness.1 When the researchers place the crystals along a line, those containing the l enantiomer leap to one side of the line, while the d enantiomer jumps the other way.
The slight catch is that to display this behaviour the crystals have to lie with a particular facet – and thus a specific crystallographic plane – on the surface. So some rather precise manipulation of the crystals is still needed to induce the separation. All the same, it’s striking to see molecular chirality generate such a difference in macroscopic mechanical behaviour.
It seems fitting that the Chinese team’s experiments involve asparagine. This was the first natural amino acid to be isolated (from asparagus juice), and it was Pasteur himself who showed in 1851 that such amino acids are optically active. It soon became clear that all those found in living systems are of the ‘left-handed’ variety: an observation given structural meaning in 1874 when Jacobus van ‘t Hoff placed Pasteur’s tentative molecular interpretation of optical activity on a firm footing with his proposal of the tetrahedral coordination around a carbon atom.
The enantiospecificity of natural amino acids, meanwhile, seemed to support Pasteur’s hunch that chirality was intimately connected to life itself: he supposed that only living things produced it at the molecular level. He wondered if this asymmetry is imposed on living matter by some external force, such as light or electrical or magnetic fields; a century and a half later, we’re still puzzled about the origin of life’s homochirality.
Serendipitous separation
Pasteur’s 1848 experiment involved a lot of serendipity. For one thing, by no means all racemic mixtures spontaneously separate when they crystallise. And he wasn’t originally studying tartrate crystals for this reason, but to investigate their water of hydration. His lab notebooks meanwhile reveal a rather messier grappling towards the truth than the ‘Eureka’ moment of revelation Pasteur and his biographers later recounted.2
Wan and colleagues see parallels with their own work, a felicitous observation that arose because the team happened to be studying both molecular chirality and dynamic crystals. Such crystals can release elastic energy and move in response to stimuli such as heat and light, for a variety of reasons such as solid–solid phase transitions.3 There is interest in harnessing this behaviour to make sensors, actuators and other devices, but the usual randomness of such motion is a hindrance.
There is a further coincidence in that it seems the jumping of asparagine crystals is due to their own hydration water molecules – what Wan and colleagues call the ‘jumping mate’, whose escape at high temperatures makes the motion, so to speak, steam-powered. (Jumps can also happen after dehydration due to conformational rearrangements of the asparagine molecules.) Because of the chirality of the crystal structure, the escape path of the water molecules has a directional bias, exerting a net force in one direction relative to the crystal orientation – a notion supported by the researchers’ molecular-dynamics simulations. The jumping is repeatable if the crystals are rehydrated.
And there’s one more bit of serendipity too. In what might be the only previous report of heat-induced jumping of chiral crystals4 (in that case the amino acid pyroglutamic acid), there was no apparent directionality to the jumps – because the mechanism (release of strain in the hydrogen-bonding network) does not recognise distinctions between the d and l forms. So Wan and colleagues, like Pasteur, chanced upon the ‘right’ crystals. But as they point out, Pasteur famously said ‘chance favours only the prepared mind’.
References
1 Y Chen et al, J. Am. Chem. Soc., 2024, DOI: 10.1021/jacs.3c13416
2. G Geison, The Private Science of Louis Pasteur. Princeton University Press, 2016
3. P Commins et al. Chem Commun., 2016, 52, 13941 (DOI: 10.1039/C6CC06235K)
4. M K Panda et al., J. Am. Chem. Soc., 2015, 137, 1895 (DOI: 10.1021/ja5111927)
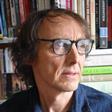
No comments yet