Philip Ball is feeling chilly
When the going gets tough, the tough get sweet. There are many physiological responses to cold conditions, from goose pimples (useless for humans, handier for hairier beasts) to the famous antifreeze proteins of fish. But one of the common strategies for insects is to fill their cells with sugar. It’s still something of a mystery why this helps.
Cold poses diverse threats to life. Ice crystals in the body can simply rupture cell walls, which is why frozen strawberries thaw to a mush. And below about -20°C, protein molecules themselves start to unravel, a process called cold denaturation. That’s not well understood yet either, although a recent paper1 suggests it involves weakening of the force between hydrophobic (water-repelling) parts of proteins that normally binds the folded form in place.
Sugars such as fructose and trehalose, as well as polyols such as glycerol and ethylene glycol, are manufactured seasonally by insects as cryoprotectants, just as we put antifreeze in our car radiators as winter draws near. Over winter, up to a fifth of the mass of some insects may consist of these substances. One consequence of cell fluid rich in sugar is simple depression of water’s freezing point. But that won’t get you very far into a bitter winter - typically, the depression will be only a few degrees, whereas the Arctic willow gall, flooded with glycerol, will survive temperatures of -66°C, so the cryoprotectants are clearly doing something else.
Surviving the cold isn’t always about not getting frozen; sometimes there’s no avoiding it, and cryoprotectants then seem to act as freeze-tolerance rather than freeze-avoidance agents. Some frogs can survive being frozen solid if they fill their cells with glucose. The sugars and polyols seem to interact with cell water to protect delicate proteins and membranes - but no one is sure how.
I got chills ...
Minoru Sakurai of the Tokyo Institute of Technology, Japan, and his coworkers have now shed some light on this through studies of the African midge Polypedilum vanderplanki,2 which survives an environmental stress with similar consequences: dehydration. Dried larvae of the midge can enter a state called anhydrobiosis, in which they show no metabolic activity but can recover viability when water becomes available. They do this by generating trehalose.
There have been two suggestions for the protective mechanism: either the water is substituted by the sugar, or the sugar promotes the formation of a glassy cell matrix rather than ice crystals. The Japanese team thinks that both are true. They find that the sugar forms hydrogen bonds with the lipids in cell membranes, replacing a shell of hydration water and preventing the membranes from becoming rigid. But the larvae also undergo a distinct glass transition as they are slowly dried. The glass is not pure trehalose, but is peppered with other components, such as proteins, that might help to disrupt crystallisation.
How, though, does a shell of sugar or polyol protect a protein when water cannot? It seems that cryoprotectants can stabilise proteins against unfolding, but whether this comes from direct protein-sugar interactions or some kind of sugar-induced modification of water structure isn’t clear. Martina Havenith at the Ruhr University of Bochum, Germany, and her colleagues recently reported signs that the latter might play a role.3 Using terahertz spectroscopy, they found that the dynamics of water molecules are disturbed a remarkably long distance away from dissolved sugars - up to about 5-7Å for trehalose and lactose. These perturbations are stronger and longer-ranged for disaccharides than for the monosaccharide glucose, which would support the notion that cryoprotection (which disaccharides do better) is tied up with the sugar’s ability to slow down the water motions and promote a pseudo-glassy state.
... and they’re multiplying
Findings by Giovanni Strambini and coworkers at the Italian National Research Council in Pisa could lend support to this idea after investigating how cryoprotectants do their job if ice crystals actually begin to form. They used fluorescence spectroscopy to study the stabilisation of a protein called azurin by sugars and polyols (sucrose, trehalose, sorbitol, glycerol) in ice-water mixtures.4 It seems none of these molecules offers strong protection against ice formation, although trehalose ‘tries hardest’: as ice appears, the protein is increasingly prone to unfold. So the cryoprotectants don’t make the native protein significantly more thermodynamically stable. Instead, the researchers think that they somehow cajole the protein to stay folded in the liquid until the whole system becomes a sluggish glass and unfolding is then simply too slow - a kinetic rather than thermodynamic effect.
So there is an emerging picture, albeit a complex one. The cryoprotectants could have a dual role. First they remodel biomolecular hydration shells, retarding water and maybe suppressing the loss of crucial protein-folding forces. Then they eventually promote the formation of a glassy matrix rather than an icy one, arresting the biomolecular structures in recoverable suspended animation. That’s clever work for a spoonful of sugar.
References
1 C L Dias et al, Phys. Rev. Lett., 2008, 100, 118 101 (DOI: 10.1103/PhysRevLett.100.118101)
2 M Sakurai et al, Proc. Natl. Acad. Sci. USA, 2008, 105, 5093 (DOI: 10.1073/pnas.0706197105 )
3 M Heyden et al, J. Am. Chem. Soc. 2008, DOI:10.1021/ja0781083.
4 G B Strambini et al, J. Phys. Chem. B, 2008, 112, 4372 (DOI: 10.1021/jp711185r)
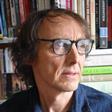
No comments yet