There has been over 60 years of changing government regulation and innovative industrial R&D in the pursuit of cleaner air: from reducing NOx and carbon dioxide emissions to developing materials for electric cars
In the 1940s, dangerously high levels of air pollution started to cause problems for several large cities, particularly in the US. This led to heavy smog, which caused poor visibility and public health problems, typified by the Great Smog of 1952 in London, UK. Scientists such as Arie Haagen-Smit at the California Institute of Technology in the US linked these new pollution problems with the use of internal combustion engines, and later showed that vehicle exhaust emissions were a major cause of the smog in the US city of Los Angeles.1 The UK and US governments responded with the first Clean Air Acts in 1956 and 1963, respectively, to regulate emissions from factories and power stations. These Acts were swiftly followed by the first regulation of car emissions, the US Motor Vehicle Air Pollution Control Act (1965),2 which set new standards for all cars in the US from 1968 onwards and required significant reductions in hydrocarbon and carbon monoxide emissions from car exhausts.3
Automotive emissions were initially reduced to meet the 1965 Act through improvements in engine tuning, but subsequent legislation placed greater reduction requirements on the so-called ‘criteria pollutants’ – oxides of nitrogen (NOx), carbon monoxide and hydrocarbons – as well as particulate matter and carbon dioxide in later years. Accordingly, research scientists within chemical companies such as Johnson Matthey (JM) and Engelhard (now part of BASF) turned their attention to innovative and more future-proof developments for car manufacturers, beginning with two-way then three-way catalysts for gasoline engine vehicles.
Two-way automotive catalysts
In the late 1960s, JM began developing commercially viable catalysts for cars that would remove hydrocarbon and carbon monoxide emissions caused by incomplete fuel combustion. The first autocatalysts were designed to oxidise pollutants within the car exhaust, converting toxic carbon monoxide into carbon dioxide (which was not regulated at the time), and hydrocarbons into carbon dioxide and water. The catalysts were made of a heat-resistant honeycomb ceramic material that was coated with alumina, rhodium and platinum. The specialised honeycomb material was developed by Corning in 1972 to support the high-temperature requirements of the catalysts. The application of precious metal chemistry was key to the success of the new catalyst technology, as these specialist materials had the highest reactivity for the required reactions. JM has specialised in precious metals since its foundation 200 years ago and has built up much expertise in sourcing and handling these materials over the decades.
The catalyst technology was initially met with scepticism until scientists proved that a Chrysler Avenger fitted with a catalyst could be driven for 26,500 miles and still comply with the 1970 US Clean Air Amendments Act – which would require a further 90% reduction in car emissions by 1975 (see Figure 1 ). These two-way catalysts became the world’s first mass-produced autocatalysts to control vehicle pollution and were fitted to vehicles in the early 1970s in the US to meet the 1975 regulations. At the time, scientists were already working on a two-way, ‘dual-bed’ catalyst4 using rhodium that could reduce NOx closer to the engine, with a downstream platinum–rhodium catalyst and airpump for oxidising carbon monoxide and hydrocarbons. The oxidation–reduction was set up in two different parts of the exhaust; the engine had to be calibrated to produce net-reducing conditions, and an airpump fitted to then create a net-oxidising environment. However, these modifications brought the disadvantages of increased manufacturing costs and decreased fuel economy.
Two-way becomes three-way
These problems were overcome by the development of oxygen sensors and the first three-way catalyst (TWC) by JM in 1979 that enabled ‘closed loop’ engine management systems. These systems could accurately balance net-oxidising and net-reducing exhaust conditions to simultaneously oxidise carbon monoxide and hydrocarbons, and reduce NOx, with a single TWC.
By 1985, JM had supplied one million catalysts
This catalyst included a cerium oxide component that could store or release oxygen in order to maintain a high conversion level for all three main pollutants. The TWC was widely adopted in the early 1980s in order to meet further tightening of US emissions limits in 1981. By 1985, JM had supplied one million catalysts to Volkswagen, and the original TWC catalyst was the prototype for today’s gasoline autocatalysts.
Three-way catalyst technology has evolved since the 1970s, but still largely comprises precious metals and an oxygen storage and release/conversion component. Over the past 30 years, they have evolved to withstand increasingly higher temperatures and to be more durable, as European legislation now requires catalysts to last between 60,000 and 200,000km. Modern catalysts, such as the alumina–ceria–zirconia mixed oxide technology,5 have been developed to operate at much lower temperatures, enabling more fuel-efficient driving. There has also been considerable development of catalyst robustness to be suitable for a wide range of operating conditions, from occasional short and low-speed trips to daily motorway driving.
Catalysts for diesel engines
Emissions standards for diesel engine cars were first introduced in 1996 in legislation known as Euro 2, by which time autocatalyst companies had already started developing diesel oxidation catalysts (DOCs) to reduce emissions of carbon monoxide, hydrocarbons and the volatile organic fraction of diesel particulate matter. The first diesel car with a DOC came to market in 1990 and its environmental impact was comparable to that of a gasoline with a three-way catalyst. The additional reliability, longevity and fuel economy of these diesel cars led to rapid growth in their popularity during the 1990s.
However, the inherent fuel efficiency of diesel engines brought fresh challenges for scientists. Less heat is generated in their exhaust pipes, meaning the catalyst needs to be effective at lower temperatures than in gasoline-powered cars. In addition, diesel engines operate under highly oxidising conditions, which is beneficial for platinum- or palladium-catalysed oxidation of carbon monoxide and hydrocarbons. But it is less suitable for reducing NOx, because most reductants react more readily with the exhaust’s plentiful oxygen than with NOx. This was overcome by developing selective catalytic reduction (SCR) systems. In these, ammonia is introduced into the exhaust and specific catalysts are used which preferentially react the ammonia with NOx instead of oxygen. This technology required adding a urea–water tank to vehicles, with the solution injected into the exhaust. The urea then hydrolyses to form ammonia, which passes over the SCR catalyst and converts NOx to nitrogen and water. In 2005, heavy duty diesel emissions legislation – Euro 4 – was introduced and SCRs became widely adopted for vehicles such as trucks and buses.
In order to further reduce NOx emissions, vehicle manufacturers have increased the amounts of ammonia added into the exhaust. This led to another challenge for the industry: how to prevent ammonia – or its distinctive odour – from coming out of the exhaust pipe. The answer was to introduce another catalyst: the ammonia slip catalyst, which oxidises excess ammonia into nitrogen. This catalyst is highly selective, to ensure that ammonia is oxidised only into nitrogen and water, not oxidised fully back to NOx.
In 2009, the Euro 5 regulations were introduced. Owing to the significant health problems associated with particulate matter and soot, these regulations included legislation that dictated acceptable emission levels from vehicles. As a result, filters began to be fitted on diesel passenger cars and heavy duty vehicles to trap soot or particulate matter and meet the new standards. Previously, the accumulated soot was removed from the filter periodically through a short burst of engine combustion that raised the temperature in the exhaust to around 600°C to burn off the soot. An alternative catalysed soot filter was developed in 1995 by JM using a nitrogen dioxide (NO2)-based reaction as part of new continuously regenerating trap technology.6,7 The system uses a platinum oxidation catalyst which converts some of the NO emissions from the engine into NO2. The gas feed then passes through a diesel particulate filter where the NO2 reacts with soot to produce carbon dioxide and nitrogen. The trap was developed as a retrofit device to control emissions from heavy duty vehicles and is still widely used today, having made a positive impact in reducing particulate pollution in many cities.
Challenges still to come
Although considerable progress has been made within the industry, there are still further challenges to overcome. The Euro 6 emissions standards from 2014 require all new diesel and gasoline cars to meet new limits for carbon monoxide, NOx and particulate matter. All diesel cars now use NOx after-treatment in addition to particulate filters to reach these regulations.
The most challenging area for emissions will be improving NOx control under the highly oxidising conditions
For diesel vehicles, the most challenging, and widely publicised, area for emissions will be improving NOx control under the highly oxidising conditions within a diesel engine. There is growing interest in technologies that enable NOx conversion at very low temperatures, such as during slow city driving. This can be achieved by using a ‘lean NOx trap’ instead of a DOC. A diesel engine typically runs under highly oxidising conditions, where NO emissions are oxidised to NO2 that is stored temporarily as a nitrate in the catalyst. Running the engine under highly reducing conditions for a very short period of time causes a pulse of reductants to arrive at the catalyst and release and convert the stored nitrate. A NOx trap therefore cycles between longer periods of oxidising conditions with short pulses of net-reducing conditions. In diesel passenger cars, this technology is usually based on platinum, palladium and rhodium chemistry that is similar to the three-way catalyst.
JM scientists have recently developed a new catalyst technology, the ‘cold start concept’, which uses a combination of metal and zeolite chemistry to store NOx immediately from engine switch-on. These materials are capable of storing NOx at room temperature, so the catalyst begins storing as soon as the engine is switched on. As the engine and exhaust heat up, the stored NOx is released and converted by the downstream SCR system to nitrogen and water. This technology enables far greater conversions to be possible over the whole drive cycle and should make a significant difference to NOx emissions control for the future.
The success of every new autocatalyst has depended on close collaboration with car manufacturers. They have continually improved the design and performance of their engines and combined these developments with catalyst system improvements to reduce the quantity of pollutants and other potentially harmful emissions. These advances also required collaboration with manufacturers of key materials, such as catalyst substrates, as well as fuel and oil companies who have adapted their products for better catalyst performance. An important example is the development of unleaded petrol, which was introduced not only for its own environmental reasons but also because autocatalysts cannot function in the presence of lead. Similarly, sulfur can inadvertently affect many autocatalysts, so fuel companies and regulators have worked together to reduce fuel sulfur levels. The catalyst innovations also required catalyst companies to have deep understanding of the regulations in order to cooperate closely with industry regulators. This has been important for deciding what kinds and combinations of catalysts would be most suitable to use, what performance features and operating conditions will be required of the catalysts, and how to develop catalysts to meet future market needs.
Beyond the internal combustion engine
Over the next few years, car manufacturers will be switching their focus to the development of electric cars. For example, Volvo announced in 2017 that it will no longer produce new solely fossil fuel-powered cars and will instead be focusing on electric or hybrid models from 2019. Several countries’ governments from the Netherlands, Norway and Germany to India propose to completely ban pure gasoline and diesel cars by 2025–2030. The UK and France plan to follow suit by 2040. For material suppliers, catalyst technology and fuel companies, these changes present new R&D challenges for the coming years, as fossil fuel-powered cars will continue to be manufactured globally for many years, but will need to comply with new emission control legislation. In addition, there will be considerable opportunities to advance current hybrid and electric car technologies, such as cathode materials for battery vehicles, to bring down costs for consumers, while increasing car battery lives.
Today’s cars emit ~1g of pollutants for every mile driven, compared with a 1960s car that emitted more than 100g per mile
Today, new materials are being developed to drive the future of electric vehicles that will ultimately succeed the internal combustion engine. New battery technologies must optimise several important aspects in order to compete with internal combustion engines: material safety, energy density, cycle life and finally cost viability. The latter is important for the successful implementation of any new material. If the battery is too expensive to make, the electric vehicles will also be expensive making public adoption minimal.
Previous battery materials have relied on large quantities of cobalt. Such materials were developed to optimise battery cycle and range properties over the past 20 years and include lithium cobalt oxide, nickel–manganese–cobalt and nickel–cobalt–aluminium materials. However, these battery technologies struggled to offer customers the driving range and the energy density that are required for the everyday use of vehicles. Furthermore, challenges associated with the supply of cobalt and the rise in global demand for battery technology has led to the increasing cost of raw materials. This means materials with high cobalt compositions are unsustainable in the long-term and alternatives are continuously being sought.
One such alternative that JM has recently developed is battery cathode material eLNO. It innovates on current battery technology and provides a material that has optimised performance characteristics to offer best in class battery capabilities. Its unique combination of elements, all considered with future availability in mind, offers enhanced energy storage and cycle life. This gives eLNO-powered vehicles superior range and longevity, currently estimated as offering a 25% improvement in both features. In addition, with lower levels of cobalt and other metals in the composition, the cost of manufacture has been reduced. These advanced materials form a platform of alternative sources of power that make adapting to any upcoming change in technology, regulation or consumer behaviour, easier.
Over the past 60 years, many governmental acts have been introduced to reduce automotive emissions and improve our air quality. As a result, today’s passenger cars typically emit around 1g of pollutants for every mile driven, compared with a car made in 1960 that typically emitted more than 100g per mile. The rapid success of this legislation can be attributed to continuous innovation and collaboration among key suppliers throughout the automotive industry. These innovations were largely led by industrial chemists who applied their expertise in chemocatalysis to the automotive industry, and collaborated with engine manufacturers, vehicle designers and fuel providers to bring new technologies to market. The automotive industry will continue to depend on chemists for further technological innovations to meet public needs for a more sustainable future, be it in the development of novel catalysts for the internal combustion engine or innovative battery materials.
Andy Walker is the technical marketing director at JM
Alan Nelson is chief technology officer and sector chief executive for new markets at JM
Note: eLNO is a trademark of the Johnson Matthey group of companies
References
1 A J Haagen-Smit and M M Fox et al,Ind. Eng. Chem., 1956, 48, 1484 (DOI: 10.1021/ie51400a033)
2 Motor vehicle air pollution control act, Public Law 89-272, US Government Publishing Office, 1965 https://bit.ly/2HoXZqV
3 Clean air act overview, US Environmental Protection Agency https://bit.ly/2lCBC7g
4 G J K Acres, Johnson Matthey, Br. Pat. 1971, 1390182
5 H-Y Chen and H-L R Chang,Johnson Matthey Technol. Rev., 2015, 59, 64 (DOI: 10.1595/205651315x686011)
6 B J Cooper, H J Jung and J E Thoss. US Pat., 1990, 4902487
7 P N Hawker,Platinum Metals Rev., 1995, 39, 2 https://bit.ly/2JDPu0s
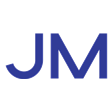
No comments yet