Philip Ball uncovers a pleasing symmetry surrounding the mysterious Casimir force
Few concepts in physical science have been made as mysterious as the Casimir force. The force, identified in 1948 by the Dutch physicist Hendrik Casimir, is the result of the quantum nature of a vacuum, which is not empty at all but is filled with spontaneously fluctuating electromagnetic fields. These can be regarded as composed of photons created when pairs of particles and antiparticles pop unheralded into existence before annihilating each other in a burst of radiation, suggesting an image of the vacuum as a seething mass of ephemeral activity.
Because the wavelengths of the photons are constrained in the space between two surfaces to be no wider than this gap, the radiation pressure is smaller in this space than in the region outside, creating the (Casimir) force that pushes the surfaces together.
Now, this does represent a valid way of looking at the situation, and does indeed make the Casimir force seem rather remarkable. But there’s another, more mundane way to understand it. All surfaces contain electron clouds that are more or less ‘floppy’ or polarisable, deformable by nearby electric fields. These clouds undergo spontaneous quantum fluctuations, and when two such surfaces are very close together, each ‘feels’ the fluctuating polarisation of the other. The result is that the two may become correlated, creating an electromagnetically induced attraction between them. This is nothing more than the familiar ‘dispersion’ or van der Waals force, which decays as the inverse-cubed power of the distance from a flat surface.
But for one surface to feel the other, a photon has to pass between them. And as the surfaces get further apart, the time taken for the photon to travel across the gap becomes non-negligible, affecting the ability of each surface to respond to the other. This changes the character of the force, specifically by making it decay instead as the inverse-fourth power of the separation. This too is then the Casimir force: a kind of ‘retarded’ form of the dispersion force.
Measuring the effect
In many ways, the second description is simpler and easier to grasp. Yet the picture based on confined vacuum fluctuations has its advantages, not least the fact that it helps us to understand a classical analogue of the Casimir effect identified in 1978 (the same year as the Casimir effect proper was first measured) by Pierre Gilles de Gennes and Michel Fisher. They recognized that fluctuations in the concentrations of a mixture of two fluids confined between two surfaces can act like the quantum fluctuations of an electromagnetic field in a vacuum: suppression of long wavelengths in the gap creates an interaction between the surfaces.
The range of this effect depends on the ‘correlation length’ of fluctuations in the mixture - crudely speaking, the distance over which a fluctuation in one part of the fluid is felt elsewhere. The correlation length gets very long - in theory, infinite - as the critical point of the mixture is approached, where the fluid is on the verge of separating into two phases. And so the interaction is important in this regime, and is called the critical Casimir force. It promises a versatile way to manipulate small particles in micro- and nanotechnologies, particularly because calculations suggest that the force can be made attractive or repulsive by varying the composition of the fluids and surfaces.
The critical Casimir force was not directly seen in experiments until the start of this year, when it was reported by Clemens Bechinger and his colleagues at the University of Stuttgart in Germany.1 They observed it by measuring the forces between a flat silica surface and a polystyrene microsphere in a mixture of water and 2,6-lutidine near its critical point. Now Bechinger’s team has shown how this force can be used to arrange such particles into ordered patterns - a genuine demonstration of the technological potential.2
The researchers have looked at the critical Casimir force between microbeads and a glass surface covered with a hydrophobic polymer film, in which an array of square islands of hydrophilic glass, 2.6 m m across, has been carved out using an ion beam. As the temperature of the system approaches the critical point, the force gets stronger and the particles are increasingly localised above the islands. In this situation, the particles feel not only perpendicular but also lateral Casimir forces from the inhomogeneous surface. At high enough particle densities, the forces act not only between a particle and the surface but also between one particle and another, promoting aggregation into clusters. This demonstration of micromanipulation using small changes in temperature is not only useful but also sets up a rather pleasing symmetry - for it was by observing the interactions between colloidal particles that Casimir, in collaboration with Dutch chemists Theo Overbeek and Evert Verwey and physicist Dik Polder, was led to postulate the quantum Casimir effect in the first place.
References
1 F Soyka et al, Phys. Rev. Lett., 2008, 101, 208301
2 C Hertlein et al, Nature, 2008, 451, 172
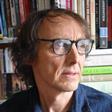
No comments yet