Philip Ball uncovers how life shepherds protons around the cell with breathtaking ingenuity
Life is pretty simple when it comes down to it. It’s a matter of shovelling stuff from one side of a wall to the other - the ’stuff’ being hydrogen ions, and the wall a cell membrane. The biochemistry that follows from this is fearsome, but, at its core, life is driven by storing up hydrogen ions and then letting them flow, just like water released from a dam.
This imbalance of protons across a membrane creates a so-called proton-motive force. It is generated by proton pumps: proteins that can actively move protons ’uphill’, against a concentration gradient. They need energy to do that, and plants get theirs by using chloroplasts to harvest sunlight. When a molecule of chlorophyll absorbs a photon, it releases an electron which hops between molecules that are successively more easily reduced, driving the proton pump as it goes. In our mitochondria, the energy is generated by reactions that break down carbohydrates.
In either case, the proton-motive force is used to power the enzyme ATP synthase, which rotates like a water wheel as it lets protons flow through, producing energy-rich ATP (adenosine triphosphate) in the process. If that’s life in a nutshell, these proton pumps clearly need to be efficient and smooth-running pieces of molecular machinery. Even so, the ingenuity that life displays in shepherding protons is breathtaking.
That life exists in water is a boon from the outset, because unlike most other liquids it can transport protons rapidly. Other small cations must physically travel from A to B, but the hydrogen ion can ’hop’ relay-style along hydrogen-bonded chains of water molecules. Rather like a Newton’s cradle, a proton hits one end of the chain and almost at once (figuratively speaking), another proton pops off the other end.
This hopping, called the Grotthuss mechanism after the nineteenth-century German scientist who proposed the basic idea, is exploited by biomolecules to shift protons. Some proteins, such as the light-powered bacterial proton pump bacteriorhodopsin, are threaded by ’water wires’: strings of water molecules that act as proton-conducting pathways.
A similar water chain also winds through the membrane protein aquaporin, which transports water across cell walls. But in this case, the chain doesn’t conduct protons - for aquaporin, letting protons through could be disastrous, as it would disrupt the delicate balance of pH and charge across the membrane. So it has to achieve the seemingly impossible feat of transporting water, but not hydrogen ions.
How it does so is still not fully clear, but one idea is that the water chain contains a defect: hydrogen bonding to the amino-acid residues within the pore forces two water molecules in the chain to sit ’back to back’ so that a proton can’t jump between them.
That would be an extraordinarily delicate feat of molecular manipulation. But it is possibly trumped by the latest revelation about why proton pumping works so well.
Magnus Brändén of Stockholm University, Sweden, and his colleagues say that there are, in effect, little proton circuits written onto the surfaces of cell membranes that help guide protons from a transporter - a pump protein - to molecules that exploit the proton-motive force, such as ATP synthase.1 The image, then, is not that of a pump spewing protons into the cell, where some gradually drift over to where they’re needed. Instead, the protons pop out of the pump’s mouth and stick to the membrane lipids, which act as proton-collecting antennae. That way, fewer ions get lost.
This idea has been mooted for years, but Brändén and colleagues have pinned it down by looking at the protonation of a single fluorescein dye molecule embedded in the walls of liposomes (cell-like assemblies of lipids).
Protonation changes the dye’s fluorescence, and so fluctuations in its brightness can be related to the rate of proton exchange with the surroundings. The researchers show that this happens at a faster rate than would be expected if protons were just being randomly exchanged with the water.
They also found that the effect depended on the lipid’s head groups - the key components of these proton circuits - being able to pick up hydrogen ions.
It’s a reminder that molecular biology isn’t just about the cleverness of proteins and nucleic acids. Even the molecules often assumed to be just part of the scaffolding, such as lipids, and the very water that bathes them all, may have inventive roles to play.
References
1 M Brändén et al, Proc. Natl. Acad. Sci. USA, 2006, 103, 19766 (DOI:10.1073pnas.0605909103)
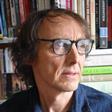
No comments yet