Unwinding protein fibrils could give a glimpse of how peptides survived on early Earth
Most biochemistry students learn that the sequence of amino acids in a protein’s polypeptide chain uniquely specifies its stable folded structure. How else can one explain the reversibility of protein folding - the fact that the chain, once opened up, can find its way back to the active globular form? As Charles Tanford and Jacqueline Reynolds put it in their masterful historical survey of proteins: ‘Sequence determines structure" became a dogma of protein science.’1
That idea remains valid, but with some crucial provisos. For one thing, correct folding of a polypeptide isn’t guaranteed in the cell, and special quality-control measures are needed to deal with misfolded versions.2 But it’s worse than that. Whereas misfolding sometimes just gives useless junk, it can also lead to the formation of stable, organised fibre-like aggregates of the rogue chains, called amyloid fibrils. These deviants first came to light through their role in neurodegenerative diseases such as Huntington’s, Alzheimer’s and Parkinson’s, where they gather into large deposits that interrupt brain function. It was initially thought that only certain proteins associated with these conditions have an amyloidogenic tendency. But, starting with the observation in 2001 that the muscle protein myoglobin will also form amyloid fibrils3 it has become clear that fibrillisation is a general property of most if not all proteins.2
In other words, fibrils represent a general alternative to the globular folded form. They are composed of polypeptide strands aligned side by side in b -sheet structures, not unlike those in silk, accounting for the robustness of amyloids. It seems that evolution has had to pick a delicate path, weeding out some protein sequences that have an especially strong propensity to aggregate into fibrils.4
Amyloid fibrils are recalcitrant, but not immune to unwinding. Cold and high pressure will dissociate them, much as they will denature globular proteins.5 But does amyloid formation have the true reversibility of regular protein folding? If so, understanding that might offer vital clues for remediating their pathological effects in the body.
Nolene Byrne and C Austen Angell at Arizona State University, US, have discovered that not only can at least one type of fibril - made from a globular enzyme - be undone but the original enzymatic activity can also be restored.6
The catch is that this doesn’t happen in ordinary aqueous solution, but in solvents called protic ionic liquids (pILs). These media are becoming increasingly valued for biochemical studies - they may, for example, enhance enzyme catalytic activity and protein crystallisation. Byrne and Angell have previously used them to study regular protein folding.7
Now they find that, while egg-white lysozyme will undergo fibrillisation in one hydrated pIL (ammonium bisulphate) when warmed to around 60oC, in some other pILs, such as ethylammonium nitrate (EAN), these fibrils will dissolve either partially or wholly. What’s more, the lysozyme recovered from EAN seems to have refolded to its active form, showing about the same activity as the enzyme simply dissolved in EAN and then diluted without fibril formation. Dissolution of amyloid fibrils has occasionally been achieved by other means, for example using concentrated solutions of the protein denaturing agent guanidine hydrochloride,8 but to recover bioactivity is very striking. It’s not exactly unscrambling an egg, but it’s close.
It’s not clear whether these solvents could be used to treat amyloid deposits in the brain. But that’s not the point. Such behaviour might offer clues about the question of how amyloid formation and stability are related to the hydration structures of the polypeptides.5 Byrne and Angell also foresee possible practical benefits: since amyloids are robust, this might offer a means of long-term storage of proteins, which could then be returned to their active state on demand.
Angell suggests an even more speculative but intriguing implication of the behaviour of proteins in pILs. One of the puzzles of how protein-like peptides might have arisen and accumulated on the prebiotic Earth is that hydrolysis would undermine the stringing together of amino acids via peptide bonds. But simple pILs such as ammonium nitrate and acetate are not only plausible prebiotic substances (they could have formed from such simple compounds as ammonia, carbon dioxide and hydrogen cyanide), but also non-hydrolysing liquids at ambient temperature with only a little water added.
Angell and his colleagues think they could have been concentrated in evaporating inland seas on the early Earth.9 Might the ability of these pILs to untangle and dissolve amyloid-like aggregates, coupled to their benign solvation properties, have offered a medium for nurturing the appearance of proto-proteins as life began?
References
1 C Tanford and J Reynolds, 2001, Nature’s robots (OUP)
2 C M Dobson, Nature, 2003, 426, 884 (DOI: 10.1038/nature02261)
3 M Fändrich, M A Fletcher, C M Dobson, Nature, 2001, 410, 165 (DOI: 10.1038/35065514)
4 C F Wright et al, Nature, 2005, 438, 878 (DOI: 10.1038/nature04195)
5 R Mishra and R Winter, Angew. Chem. Int. Ed., 2008, 47, 6518 (DOI: 10.1002/anie.200802027)
6 N Byrne and C A Angell, Chem. Commun., 2009, DOI: 10.1039/b817590j
7 N Byrne and C A Angell, J. Mol. Biol., 2008, 378, 707 (DOI: 10.1016/j.jmb.2008.02.050)
8 B A Vernaglia, J Huang and E D Clark, Biomacromolecules, 2004, 5, 1362 (DOI: 10.1021/bm0498979)
9 N Byrne et al, Aust. J. Chem., 2009
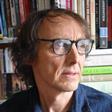
No comments yet