If DNA polymerisation is reversible, asks Philip Ball, why don't we end up with some static equilibrium?
Erwin Schrödinger’s seminal little book What Is Life? (1944) is usually remembered for its uncanny prescience in attributing the genetic basis of heredity to a molecular ‘aperiodic crystal’: an entity that, while lacking a truly periodic arrangement of atoms, somehow encodes information and protects it from the decay that the Second Law of Thermodynamics seems to threaten.
Schrödinger was deeply puzzled by how the structural precision and stability that seemed to be required of genes seemed at odds with the prevailing notion that molecular science was statistical, pervaded by randomness. The key, he suggested, was that ‘living matter evades the decay to equilibrium’ by extracting order from its environment, ‘to compensate the entropy increase it produces by living’. That trick is in turn made possible by metabolism, the generation of energy from environmental sources. Starting from the orderliness of the ‘aperiodic crystal’, biological systems use energy production to generate ‘order from order’.
Schrödinger intuited that the explanation must be bound up with the details of the molecular machinery of genetic translation and replication, and would therefore be answered not by physicists but by biochemists. That is pretty much the conventional view today: enzymes use DNA as a template to make more, identical DNA, while this DNA encodes the molecular devices needed for its own propagation.
But David Andrieux and Pierre Gaspard at the Free University of Brussels in Belgium have now offered a new perspective on Schrödinger’s dilemmas. They draw on the legacy of Ilya Prigogine, the late founder of the ‘Brussels school’ of research on nonlinear and complex systems whose work in this field earned him the 1977 Nobel prize in chemistry.
Prigogine’s seminal work on the behaviour of systems out of thermodynamic equilibrium, beginning in the 1940s, was itself founded on the earlier contributions by physicist Lars Onsager, a pioneer in statistical mechanics. In the 1930s, Onsager considered how processes that are only slightly out of equilibrium generate entropy. So at least some of the ingredients that Schrödinger was grasping for in his book - the relationship between the disequilibrium caused by metabolism and the ‘economics of entropy’ in life - were already available when he wrote it.
Andrieux and Gaspard, however, bring to this question another ingredient: information, which was itself being couched in the language of statistical mechanics in the late 1940s by Claude Shannon at Bell Laboratories. Shannon had in fact already started to think in 1940 about how digital arithmetic might be applied to an understanding of genetics.
Andrieux and Gaspard say that the transcription and replication of DNA is not really about the generation of order, but of information, which is stored spatially in the sequences of RNA or DNA templated from the parent strand. There is a tendency to regard this as a deterministic affair: the sequence of the new strand is fixed by that of the template according to the standard pairing rules for nucleic-acid bases. But of course copying errors do happen. Some get corrected in ‘proof-reading’ steps, but they need to be kept rare at the outset. The two researchers argue that this is not simply a matter of equilibrium chemical kinetics for the base-pairing reactions, but depends crucially on the fact that the polymerisation is a non-equilibrium process with an intrinsic propensity to generate information.
The authors show that the fraction of errors in a non-equilibrium templated polymerisation like this decreases sharply as the enthalpic driving force increases. When they put into their equations some figures for the enzyme-driven polymerisation of human mitochondrial DNA, they find a sharp threshold: above some critical driving force big enough to take the system far out of equilibrium, the error rate plummets, while both the speed of replication and the amount of information shared between the parent and copied strands reach maximal plateaux. Only in this regime can the copy mine the template efficiently for information.
To put it another way, molecular information can only be stored and retrieved reliably away from equilibrium. Two issues add to the intrigue. First, the theory works also for non-templated polymerisation, suggesting how a DNA-like polymer could acquire information from its environment in non-equilibrium conditions: a situation relevant to the origin of life. Second, this process turns out to be irreversible: to have a preferred direction in time. As a result, say the authors, ‘biological systems are physicochemical systems with a built-in thermodynamic arrow of time’.
References
D Andrieux and P Gaspard, Proc. Natl Acad. Sci. USA, 2008, 105, 9516 (DOI: 10.1073/pnas.080204910)
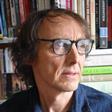
No comments yet