Enzymes have evolved into nature’s reaction facilitators over millions of years and their unique catalytic abilities drive science by connecting essential life elements
Transitioning to greener chemistry and more sustainable manufacturing processes are among the pharmaceutical industry’s biggest challenges. With the global population expected to exceed nine billion by 2050 – increasing demand for finite resources – it’s vital that manufacturers boost sustainability and reduce the environmental impact of their processes. Another challenge to the pharmaceutical industry is the increasing size of the elderly population. A World Health Organization report on global health and ageing projects a growth in the number of people aged 65 and over from the estimated 524 million in 2010 to nearly 1.5 billion in 2050. To accommodate this expanding and ageing population and meet lower, or net zero, carbon goals, the pharmaceutical industry needs cleaner, less wasteful technologies. That approach is vital to meeting the environmental, economic and social demands of future medication.
Over the past decade, many pharmaceutical companies have opted for green chemistry practices for drug discovery, development and manufacturing. This shift is being driven by the realisation that greener processes not only offer an environmental advantage but can be cheaper with less waste – providing a competitive edge.
Nature uses the life elements to orchestrate reactions that produce molecules of incredible complexity
Enzyme-based catalysis has emerged as an innovative technology for chemical manufacturing that meets many of the core green chemistry principles. By developing and applying these technologies in the pharmaceutical industry, we can create significant and positive environmental change. JM, an applied science and sustainable technologies leader, is committed to applying its extensive bio- and chemo-catalysis capabilities to help build effective processes for the pharmaceutical and fine chemicals industries.
The natural way
In recent years, the drug discovery process has shifted towards increasingly complex active pharmaceutical ingredients (APIs). These compounds often require multiple synthetic steps, greatly lowering the atom economy of their associated processes. Additionally, these processes are carried out in organic solvents, with large quantities of chemical reagents in the form of oxidants, reductants, and acids and bases. Using these chemicals means high waste levels and costly waste treatment processes. These factors have led to an increased desire for alternative technologies that can deliver effective, safe and cost-effective drug development and manufacture. Consequently, nature’s approach to chemical innovation sparked the pharmaceutical industry’s interest.
Throughout nature, biochemical systems undertake an enormous variety of chemical reactions that are catalysed predominantly by enzymes. The simple and abundant elements that we associate with life – carbon, hydrogen, oxygen and nitrogen – are used by nature to orchestrate reactions that produce molecules of incredible complexity. These vital molecules include lipids, carbohydrates, nucleic acids and other proteins.
Like all catalysts, enzymes increase the rate of a reaction by lowering the activation energy. However, one advantage of biocatalysts over chemical technologies like chemocatalysts include their ability to function under mild reaction conditions – as well as their low physiological toxicity and environmental impact. In pharmaceutical manufacturing, these advantages translate to reduced operating costs and waste, as well as simplified production routes. The most desired properties of biocatalysts include their high levels of stereo-, regio- and chemo-selectivity. Compared to traditional chemocatalysts, enzyme catalysts often reach much higher levels of selectivity. This makes them an invaluable technology for the asymmetric synthesis of chiral intermediates. The importance of chirality within the pharmaceutical industry is well-documented and current research estimates that nearly 95% of drugs will be chiral by 2020.
For drug manufacturers, the ability to consistently and reliably produce one enantiomer is crucial
Limonene is a relatable example of the dramatic effect chirality can have on a molecule. A naturally occurring hydrocarbon found in citrus, limonene’s structure has a chiral centre. Like many other flavour and fragrance molecules it is found in nature as two enantiomers, (R)- and (S)-limonene. Although these molecules hold identical physical properties, the difference in chirality can lead to different biological properties. In this case, (R)-Limonene is the isomer that contributes to the smell of oranges, whereas (S)-limonene has an aroma similar to turpentine or lemon. For drug manufacturers, the ability to consistently and reliably produce one enantiomer is crucial. This is because often only one of a drug’s enantiomers is responsible for the desired physiological and therapeutic effects – while the other enantiomer is less active, inactive or even harmful. Enzymes provide manufacturers with an effective technology to produce optically pure drugs.
Tomorrow’s biocatalysts today
Despite the clear benefits of the application of biocatalysts for the synthesis of chiral intermediates, enzymes have only recently been applied within industrial processes. This is because enzymes have evolved to be highly specific to the environments and reactions which they catalyse. When it comes to applying these enzymes in industrial processes, the high substrate concentrations and variable temperatures often prove unsuitable for biocatalysts, resulting in low selectivities, low activities and low yields. Additionally, enzymes are commonly denatured by organic solvents. This can greatly impact processes where enzymes are applied as part of a multi-step process with chemical reactions and catalysts.
In recent years, advances in computing technology, biotechnology and sequencing have fuelled advances in protein engineering. Directed enzyme evolution and rational design are now widely applied by biological engineers to produce enhanced, high-performing enzymes that can work in a broader range of conditions. Given the complexity of the sequence – structure – function relationship of enzymes, protein engineering has largely relied on random mutation, screening and selection – performed iteratively over multiple rounds – to obtain a suitable final biocatalyst fit for purpose. While there are many advantages to this technique, the process can be time-consuming. In fact, it can take up to a year to find and alter a wild enzyme into an optimised biocatalyst for industrial application. The primary cause is the painstaking process of screening the exponential numbers of enzyme variants created in each round of mutagenesis.
To accelerate the enzyme optimisation process, JM scientists leveraged the company’s expertise in computational modelling, catalysis development and manufacturing to devise cutting-edge enzyme engineering technologies.
Biocatalysis transformed from a peripheral technology to an industrially practical technology over 50 years
JM’s computational technologies combine advanced software tools and large databases to rapidly predict the best existing natural enzyme for a desired chemical reaction or process. In cases where an ideal natural enzyme cannot be found, JM turns to enzyme engineering. But instead of the widely used directed evolution approach, JM leverages its smart library design. In this process, thousands of enzyme variants are computationally screened by analysing the changes in their amino acid sequence in the context of their encoded 3D structure. Subsequently, libraries of enzyme variants are created, with the latest molecular biology techniques, produced and extracted from E. coli for testing in their ability to catalyse the desired reaction or process. Although this method drastically reduces the number of enzyme variants that need to be screened, the number can still be in the range of tens of thousands of enzyme variants. High-throughput material handling and analytics are vital to speed up the process.
The future of biocatalysis
It is important to realise that biocatalysis and chemocatalysis are complementary technologies. When efficiently applied to the pharmaceutical process, these technologies can combine to greatly improve the drug manufacturing process, both economically and environmentally. When designing new processes for innovative APIs, it is important that pharmaceutical companies collaborate with experts in both of these fields. JM’s ability to leverage its wide-ranging catalysis expertise and manufacturing capabilities allows for faster and deeper integration of these technologies into manufacturing processes.
The impact of biocatalysis within the pharmaceutical industry continues to grow. Over the last 50 years, driven by chemical, biological and technological innovation, biocatalysis has transformed from a peripheral technology to a mature and industrially practical technology. The future of biocatalysis technology depends on our ability to rapidly, economically and efficiently engineer, or even design, enzymes for industrial processes. In applying its scientific expertise to industry need, JM is driving the development of next-generation enzyme technologies to enhance quality of life and to create a more sustainable future.
Additional information
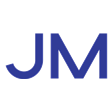
No comments yet