Understanding why nature's materials are so smart could be the first step to educating our own dumb polymers, argues Philip Ball
To make everything from bullet-proof vests to silly putty, we currently produce several million different formulations of industrial polymers, the vast majority based on petrochemicals. And yet to make an astonishingly wide range of ’advanced’ materials, including high-quality lenses, superstrong fibres and durable adhesives, nature uses just two types of polymer (polypeptides and polysaccharides), ultimately made from air, earth and sunlight.
I know this sounds like the lead-in to the familiar refrain that says ’isn’t nature wonderful, and why don’t our technologies follow its example?’ But the debate about the virtues and limits of biomimicry is often hamstrung by its reliance on anecdotes - there hasn’t really been a good way to think about the subject in a systematic manner.
Julian Vincent at the University of Bath has now proposed just such a system, based on the ’theory of inventive problem solving’. Known by its Russian acronym Triz, it was devised half a century ago by the Russian writer Genrich Altshuller and brings to light some striking contrasts between the technical solutions favoured in engineering and those found in nature.
Triz sounds a little eccentric at first encounter. Its central tool is the ’contradiction matrix’, a table that lists all the generic obstacles that arise in problem-solving, posed in terms of a nexus of two conflicting requirements. For each of these ’conflict pairs’, the Triz matrix lists the most common solution. So when you run up against a problem of design, you simply identify the relevant conflict in the matrix and look up the answer.
For example, you want to make a structure that is stiff yet flexible. One answer is to compartmentalise: use stiff components hinged together, like a suit of mediaeval armour. Another solution is to make the material continuous yet softer along the hinge line, which might give you something like insect cuticle.
Vincent and his colleagues have prepared Triz matrices for conventional engineering and for biology and compared the two. Rather than trying to make sense of the 39 x 39 matrices in each case, they condensed the solutions into six very broad classes: composition, structure, spatial arrangement, time, energy and information.
The conclusions are striking. First, there was only a 12 per cent degree of similarity between the two matrices, suggesting that nature usually has quite different problem-solving strategies to those of engineers. Second, the engineering solutions vary at different size scales. From nanometres to millimetres, engineers almost always manipulate energy, changing its source or type. Put crudely, if you have a small-scale problem, you throw energy at it. You want to join things together? Weld them. You want to move things? Stoke the boiler. You want to break things down? Cook them.
At larger scales, ’structure’ becomes gradually more significant than ’energy’ for engineers. But in biology, the proportions of each solution class stay more or less constant at all sizes, from nanometres to kilometres. And crucially, they commonly involve manipulating information, not energy.
We know that DNA holds the blueprints for proteins, but somehow that information remains relevant far beyond the molecular scale so that all biological matter is ’informed matter’. Chemistry is the key to this because ultimately that’s where the information resides. Using ’information’ in biology is then a matter of manipulating the shape and combinations of just a few materials, at very little energetic cost. Vincent and his colleagues therefore argue that ’instead of developing new materials each time we want a new functionality, we should be adapting and combining the materials we already have’.
This might solve the recycling problem. There are just five or six plastics that can be separated efficiently for recycling, yet between them, they offer just about the whole range of properties that known polymers exhibit - we don’t really need the others (except for patents and branding). In nature, recycling is an essential part of the equation - ’if you didn’t have it, you wouldn’t have us’, Vincent says - and it is easier because there are so few polymers to think about.
A popular objection to biomimetics is that we’ve got bigger problems to solve than nature has. Nature doesn’t have to stop space shuttles from burning up, for instance. But Vincent points out that while we’re fixated here on resisting heat, the biological Triz matrix can offer other solutions, such as having sacrificial layers. And indeed, the Russians heeded that, using disposable wood to shield some of their spacecraft on re-entry. The message is that if nanotechnology is to be truly transformative, it should be biomimetic at least to the extent of incorporating information as a key parameter. Once we do that, we won’t need lots of new materials - we can just be more inventive with the ones we’ve got. That, Vincent admits, ’challenges engineering at its roots’.
References
J F V Vincent, et al, J. Roy. Soc. Lond. Interface, 2006, 3, 471 (DOI:10.1098/rsif.2006.0127)
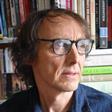
No comments yet