It’s worth paying attention to biologics – they promise targeted treatments for a range of conditions, if we can overcome the technological challenges
The desire to avoid both the side-effects associated with some small-molecule therapeutics and invasive surgical treatments has led to research focused on targeted therapies. Much of this research is now focused towards the field of biologics, which promises targeted treatments for a range of conditions. For the promise of many of these therapies to become a reality, however, technological challenges must still be overcome, with many exciting novel treatments currently on, or making their way to, the market.
Biologics are one of the fastest growing classes of therapeutic compounds, rapidly outpacing the growth of small-molecule drugs. By 2020, analysts are expecting biologics to make up over a quarter of the entire pharmaceutical market. This drive towards biologics is due to their ability to reach targets considered ‘undruggable’ using small-molecule therapies, and because of the benefits, or rather the reduced side-effects, offered to patients through better-targeted therapies.
Twentieth century pharmaceutical research focused on medications constructed from small molecules. But the last decade has seen the profitability of traditional, small molecules decline, and with fewer drugs offering the ‘blockbuster’ potential returns enjoyed in the recent past, drug development is becoming harder and more expensive. Medicines are now more commonly focused on smaller, more homogenous patient groups rather than the ubiquitous pharmaceutical products known to treat a multitude of diseases and illnesses.
One such area where small molecule medication is common is in the treatment of cancers. However, cancer chemotherapy is notorious for its harmful side-effects. This is the result of the often-indiscriminate nature of small-molecule treatments, affecting normal healthy cells as well as the target tumour cells. These issues cause major concern for both patients and physicians, and tolerability has limited the use of more potent cytotoxins. Research has therefore been performed into alternative, more targeted, small and large molecule therapies.
Biologic treatments, as the name suggests, are derived from biological processes – for example, copying an individual’s ability to grow or develop antibodies. Instead of being chemically synthesised, biologic pharmaceuticals are manufactured and harvested directly from the cell’s biological processes. As a result, their structures are much larger and more complex. Consider the comparison, for example, of aspirin, which consists of only 21 atoms, to Herceptin – an anti-cancer monoclonal antibody (an antibody manufactured by clones of a biological cell) – which consists of something in the order of 20,000 atoms. Biologic treatments can excel in targeting receptors and proteins that cannot be achieved using small molecule therapies and potentially achieving more potent interactions with the drug target, or with less potential for off-site interactions.
Challenging production from start to finish
The potential offered by biologic drugs can only be realised if certain industrial challenges associated with their development, and manufacture can be properly addressed. For the biopharmaceutical industry, a drug’s commercial success relies not only on whether the costs of discovery, research and development can be recouped, but also whether an efficient manufacturing solution can be achieved at the relatively small scales required for many biologic treatments. The challenge is especially acute when considering the high investments that are typically required to create a safe and efficient biologics manufacturing plant. It is also important that a solution is suitably ‘scalable’ as the new treatment progresses through clinical trials and to commercialisation, and all this is set against the ever-present challenge of securing regulatory approval. Outsourcing is an obvious solution for many innovators, as contract development and manufacturing organisations (CDMOs) can often achieve greater efficiencies across multiple customers and programmes. Many have proactively invested in technologies appropriate to relatively small-scale production, including single-use biomanufacturing processes.
As discussed previously, biologic molecules are large, complex structures requiring extensive analytical data. Characterisation of these molecules through conventional methods is difficult owing to this structural complexity. Analytical technology is moving on at a pace, which is just as well considering it is another important factor in the development and approval of better biologics. State-of-the-art techniques that prove the activity, stability and reproducibility of biologics are essential if the increasingly strict demands of regulators are to be met. The relatively recent licensing of the first biosimilar in the US – almost a decade after biosimilars were first commercialised in Europe – has helped increase the demand for analytical services, as rigorous analysis is a vital part of the process to prove biosimilarity and satisfy regulatory authorities.
As industrial understanding of the biological technology improves, streamlining the development process, the overall development costs and timing can be improved. More advanced methods of flexibly creating cells lines, such as Catalent’s GPEx technology, provide rapid, reliable methods that facilitate the production of biologics more efficiently. Ultimately this provides a faster method of advancing clinical studies. Successive technological and pharmaceutical innovation will make affordable, more efficacious biologic treatments commonplace in the future.
ADCs: combining biologics with molecular chemistry
The two fields of potent small molecule treatments and biologics are not mutually exclusive. Smart, designed chemistry can link the two fields to deliver effective oncology medicines. Chemical and biological research has focused on combining the advantages offered by the two different fields into one, highly potent, class of drugs known as antibody–drug conjugates (ADCs).
ADCs are one of the most advanced oncology treatments to have been developed in recent years, and they were conceived as targeted cancer treatments. All ADCs are constructed from three individual components: an antibody scaffold; a chemical linker; and a highly cytotoxic molecular payload. By using the ability of antibodies to couple specifically to certain proteins known as antigens, a triggered release of the cytotoxic molecule within the desired cell can be achieved, mitigating off-site cytotoxicity that may lead to unwanted side-effects.
As of 2017 there are four ADC drugs that have received approval from the US Food and Drug Administration, with more than 60 others currently under development.1 The first generation of ADCs, developed in the 2000s, encountered several design flaws that prevented extensive use. But these issues have now been remedied using the most advanced biochemical processes. The subsequent generations of ADCs show promise as more active cancer treatments and overcome many of the safety concerns.
A unique construction
The construction of an ADC is one of the fundamental challenges in the field, requiring innovative chemistry and advanced understanding of biological properties. There are various technical and chemical challenges associated with each component that must be overcome to deliver the most efficacious drug.
The largest component of any ADC is the antibody scaffold. Effective design will identify an antibody that is tailored to a specific antigen associated with tumours. The difficulty is in the tumour-specific antibody identification process that, upon administration, will seek out its associated antigen. An appropriate antigen must be found that is prevalent in tumour cells and rare in healthy cells.
The major component of an ADC is its small molecule ‘warhead’ that delivers the toxicity necessary to destroy tumorous cells. Molecules with sufficient cell potencies in isolation might not be sufficient when coupled with an antibody. In fact, early ADC therapies showed disappointing activities, thought to be a result of linkers that were unstable in plasma releasing highly cytotoxic drugs too early, or because of the low potency of the cytotoxin.2 Nowadays, the push is towards finding more cytotoxic molecules. Attempts to deliver chemotoxic drugs such as doxorubicin or paclitaxel via ADCs continually results in poor efficacy because the drugs do not reach lethal concentrations in tumours.3
A linker that is appropriate for the application then needs to join the antibody and small molecule moieties. If a linker is too weak, the cytotoxic molecule will be released during transportation in the bloodstream prior to localisation in the target cells, disrupting the site-specific advantages associated with using antibody–antigen coupling. Early ADCs suffered from linker instability leading to this premature release. Subsequent generations of ADCs, which feature more innovative chemistry, incorporate more advanced linkers that address this pitfall.
Linkers can be broadly split into two categories: cleavable and non-cleavable. Cleavable linkers respond to the unique conditions inside the target tumour cell. Upon subjection to these appropriate conditions, the release mechanism of the cytotoxic molecule is triggered. Non-cleavable linkers release the toxic molecule upon degradation by a biochemical catabolic mechanism that breaks the ADC down into its individual components. The goal of both linker designs is the release of the cytotoxic molecule only within the desired cell, hence delivering targeted cytotoxicity.
The necessity of smart chemistry
Combining all the components of an ADC together as a simple ‘one-pot combination’ of antibodies, linkers and molecules is not sufficient to create the most efficient ADCs. Early ADCs suffered from poorly controlled drug-to-antibody ratio (DAR), owing to the non-specificity in which the linker and small molecules were bound to the antibody. This effect is known as ADC heterogeneity. This creates problems in quality assurance and control in manufacturing and, if unchecked, could have a detrimental effect on a drug’s capabilities due to the variable toxicity and potency between drug batches, meaning treatments have unpredictable results.
The goal now is the creation of homogeneous ADCs where the DAR can be better controlled. This can be achieved using designed, smarter chemical synthesis, leading to more efficacious medication. One such process has been developed at Catalent Biologics, and is now known as the SMARTag (specific modifiable aldehyde recombinant tag) technology. This method is designed to produce site-specific homogenous antibody–drug conjugates.
First, batches of antibodies are manufactured that are modified to contain a specific amino acid sequence. Insertion of the amino acid sequence is possible through cell line cloning techniques and can be directed to any desired point on the antibody with minimal structural distortion. This amino acid sequence is converted to an aldehyde functional group by a specific enzyme during antibody production within the cell. The external aldehyde tag is then used as a functional group ‘handle’ to direct site-specific linker addition. This approach allows for the possibility of precise payload positioning and control of the drug loading.
Next, using aldehyde-specific chemistry, the linker and pre-bound small molecule compound can be coupled directly to the unique aldehyde handles on the antibody. Due to the specific insertion of the amino acid sequence, the number of aldehyde handles has been controlled allowing the DAR to be uniform between batch synthesis. To remain stable in the body, the most desirable linker–antibody bonds involve carbon–carbon bond formation. To achieve this, Catalent’s proprietary HIPS (hydrazino–Pictet–Spengler) ligation is used to create products with proven long-term stability in the bloodstream.
Following construction, the ADC can now be administered into the body. Using the specificity of the antibody–antigen coupling, specific tumour cells will be targeted. Once the antibody is bound to the antigen, the ADC undergoes several cellular processes that mediate cell entry. Once inside, lysosomal proteases degrade the ADC into its individual components, cleaving the HIPS link. Since this process occurs within the cell, the release of the cytotoxic compound is contained. Cell death is then mediated via the relevant processes of the chosen molecule and antibody. This approach to optimise ADC design has yielded therapeutics demonstrating significantly improved safety and efficacy profiles in preclinical studies vs. first generation approaches.
Biologics are worth the wait
Years of pharmaceutical research have led to this new era of treatments. The advent of newer technologies and the deeper understanding of the pharmaceutical processes these provide will always lead to newer, more efficient drugs.
One of the largest applications of biologics is as an oncology treatment. Increasingly, the cost to discover, develop, approve, manufacture and market more advanced biopharmaceutics is being weighed against the cost of treating a patient with cancer, and there is a natural pressure to consider ‘quality of life’. Further research into the technological processes and the science behind the drug, and the processes used to manufacture them at an appropriate scale, will allow more therapies to become available.
Biologics will continue to show robust growth for decades to come. The field of biologics is representative of a wider shift in focus away from ‘one size fits all’ treatments and towards more targeted medications to treat specific groups of patients. For service providers, there are a multitude of opportunities to provide new avenues for biologic-based treatments. Outside of oncology, biologics are already being applied to fields such as chronic diseases – for example autoimmune diseases – and infectious diseases. Biological pharmaceuticals will advance with each novel scientific innovation, ultimately leading to newer, more efficient treatments for patients.
Mike Riley is the vice-president and general manager of Catalent Biologics
References
1 A Beck et al,Nat. Rev. Drug Discov., 2017, 16, 315 (DOI: 10.1038/nrd.2016.268)
2 A Ritter,Pharm. Technol., 2012, 36, 42 http://bit.ly/2FK7amq
3 S Jaracz et al,Bioorg. Med. Chem., 2005, 13, 5043 (DOI: 10.1016/j.bmc.2005.04.084)
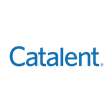
No comments yet