Muonium spectroscopy can reveal photochemical reactivity and dynamics of specific carbons within an organic molecule
A new technique involving an exotic antimatter analogue of the hydrogen atom can disclose the photochemical reactivity and dynamics of specific atomic positions within the carbon framework of organic molecules. The method can be used to explore light-induced behaviour in molecules used for making organic photonic devices such as light-emitting diodes, and might eventually offer insights into the fundamental processes underpinning biological photochemical reactions such as those in photosynthesis.
It is a modification of the well-established method of muon spin-rotation spectroscopy (μSR), in which the response of a muon’s spin to an electromagnetic signal offers a probe of the particle’s local environment. In the new technique, developed by Alan Drew and Peter Heathcote of Queen Mary University of London and their co-workers, positive muons – the antimatter counterparts of the electron’s heavy cousin – mimic hydrogen nuclei, capturing an electron to form a hydrogen-like muonium atom which can insert itself onto an unsaturated carbon atom. The muons survive for only a few microseconds before decaying into positrons and neutrinos, but that’s long enough to disclose something about their environment and the changes in it induced by photoexcitation.
The researchers used muons produced at the ISIS Muon Facility of the Rutherford Appleton Laboratory in Didcot, UK. They first exposed their samples – solutions of a pentacene-based organic semiconductor abbreviated to TIPS-Pn – to a pulse from a green laser, creating an excited state. This excitation altered the reactivity of carbon atoms in the aromatic rings, for example towards reactions with muonium atoms to form neutral radicals. By altering the time interval between laser excitation and exposure to a subsequent pulse of muons, the researchers could probe how this photexcitation decays over the course of several microseconds.
Pinpointing reactivity
There are four distinct positions on the rings at which the muonium might attack – and each has a different reactivity. The researchers could look at each position in isolation by applying a magnetic field to the sample. Thanks to the coupling between the muon spin and the electron and nuclear spins in its immediate vicinity, the muon spin has different energies in different orientations and can be flipped by absorbing radiofrequency radiation at a frequency specific to each of the four spatial positions. The amplitude of this resonance depends on the kinetics of muonium attachment – that is, on the reactivity of the carbon atom at the particular location being probed. By tuning the frequency of the radiofrequency ‘probe’ pulse, these kinetics can be deduced for each of the four types of carbon atom in turn. The results show that the laser pulse creates a photoexcited molecule that is most reactive on the ‘sides’ of the two outermost aromatic rings of the pentacene core.
Donald Fleming, a specialist in muon chemistry working at the TRIUMF accelerator facility in Vancouver, in Canada, says that this is the first time electronic excited states have been probed by this kind of muon technique, and that the timescales of muon processes make the method well suited to studying such short-lived free radicals. However, he feels that at this stage the data only marginally support the claim to distinguish reaction kinetics for the four different sites on the molecules. ‘It may be possible to get the level of information advertised in the present paper if sufficient statistics were taken to render the results more believable,’ he says. But extracting such information for complex molecules such as biomolecules will be even more challenging, he cautions.
Drew agrees that ‘it is very challenging to get the reaction kinetics from a single concentration of solute’, as done here. He says that this would be easier if measurements were made at different temperatures and concentrations – an experiment the team is now planning.
Probing such processes in biochemistry might remain very difficult for now. ‘I don’t think we will ever work on proteins,’ Heathcote says, ‘but we have already commenced studies on amino acids.’ He adds that it should be possible to use the technique to follow photoexcited electronic processes in solid-state organic semiconductor devices.
References
K Wang et al, Nat. Mater, 2016, DOI: 10.1038/nmat4816
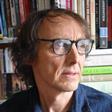
No comments yet