The history of the surprisingly simple metal complex that is still the gold – or should that be platinum? – standard in cancer drugs
Any discussion of anti-cancer treatments now frequently touts the emergence of immuno-oncology and biologics as the next generation of cancer pharmaceuticals. In fact, some of the most exciting new research has focused on delivering targeted medications that can specifically interact with, and deliver potent molecules to, tumour cells. But treatments such as antibody drug conjugates – one of the fastest growing classes of oncology therapeutics – are still relatively new fields, with only four currently available on the market and many others remaining in clinical trials. Pharmaceutical research into newer, more effective medications has long been garnering interest from academic researchers and gaining extensive industrial backing.
It might come as no surprise that cancer is the leading cause of death in the developed world. In 2015, 8.8 million people died from causes attributed to cancer, accounting for almost one in every six deaths worldwide. As with every illness in modern society, extensive industrial and academic research has set about to answer the questions of how cancer can be detected, prevented and most importantly, cured. For such a morbid topic, it should hearten readers to learn that as modern treatments and medications have improved, the rate of cancer mortality has declined. From its peak in 1991, the cancer death rate has dropped by 26% as of 2015, a testament to the wonders of modern medicine.
One simple metal complex revolutionised the treatment of cancer in the latter half of the 20th century
Let us now take a step backwards in time, to the discovery of platinum-based anti-cancer drugs that revolutionised the treatment of cancers. This is the story of cisplatin, from the accidental discovery of its anti-tumour properties in 1965 to the related generations of platinum-based derivatives. This is the story of how one simple metal complex revolutionised the treatment of cancer in the latter half of the 20th century.
The original: Cisplatin
Cisplatin is an example of a metal complex. The name itself is a merger of information referring to the stereoisomeric geometry adopted, cis-, and the platinum metal at the heart of the complex, -platin. Its formula is cis -PtCl2 (NH3)2, with the platinum metal centre bonded to four ligands: two ammonia (NH3) and two chloride (Cl) groups. What makes cisplatin special is the platinum metal, which enforces the adoption of a square planar geometry, meaning all four ligands are in the same plane as the metal centre. There are two possible stereoisomers that a PtCl2 (NH3)2 complex can adopt, cis and trans, which differ according to the geometric arrangement of the four ligands. Later investigations would show that the cis configuration is essential for the anti-tumour activity.
It might shock readers to learn that cisplatin dates back to 1845, when it was originally known as Peyrone’s chloride. But its anti-tumour properties were only discovered in the 1960s, in what could be considered one of the greatest accidental discoveries in modern science.
Like all scientific investigations, it all originates from a hypothesis. In the early 1960’s, physicist-turned-biologist Barnett Rosenberg at Michigan State University in the US hypothesised that applied electric or magnetic fields might affect cell division. He set about testing the effect of a field on the growth of Escherichia coli, using an experimental set-up where the field is supplied through a series of electrodes. Luckily for him – and incidentally humanity – the setup included an electrode set made from platinum. After turning on the field, Rosenberg noticed something odd about the bacteria. They were around 300 times longer than expected.1 Following a detailed analysis, the cause of the bacteria elongation was determined to be caused, not by the presence of the field, but by the presence of two platinum-based complexes which had prevented bacterial cell division. One of which later became known as cisplatin.
His attention then quickly turned to human biology. If cisplatin inhibited cell division seen in the bacteria, what effect would it have on tumour cells? With a hypothesis formed, Rosenberg set about with the experiment. Results published in Nature in 1969 showed that cisplatin caused marked tumour regression in mice.2 The first steps towards an effective metal-based treatment for cancer had begun.
The story of cisplatin continued through the 1970s, with the drug entering clinical trials in 1971 before being made commercially available in 1978. As with any pharmaceutical discovery, shortcomings had to be addressed relating to how the drug was administered, but this was a major advance in a field that had seen survival rates estimated to be as low as 10% in testicular cancer. The advances in cancer treatment offered by cisplatin cannot be understated, as this simple drug revolutionised survival rates of many cancers. Typically prescribed for ovarian, lung and stomach cancer, it was actually in the treatment of testicular cancer that survival rates saw the largest improvement. Some estimates have suggested that cisplatin resulted in a survival rate increase from 10% to upwards of 85%.3 Cisplatin is still used to this day, both as an anti-cancer treatment, and is cited as the ‘gold standard’ of cancer drugs against which all newly proposed drugs are measured.
The mechanism and exact biological reasoning for cisplatin’s anti-cancer properties is still under investigation and is subject to debate. The advent of more powerful research-enabling technologies and the scientific community’s increased knowledge of cellular processes have resulted in refined understanding of the mechanisms behind cisplatin’s anti-tumour properties.
Following an intravenous injection of cisplatin – which is the only way to administer the treatment – cisplatin is transported around the body in its neutral, relatively unreactive form. For cisplatin to be reactive, it first must be activated. Following entry into a cell, cisplatin’s activation is achieved through water replacing its chloride ligands. The complex is then positively charged, which facilitates the interaction with the negatively charged DNA backbone. This ultimately leads to covalent binding with specific sites on the DNA base pairs through an exchange of the weakly bound water ligands. There are several plausible methods of binding, forming bound complexes known as cisplatin–DNA adducts that distort the helical structure. It is this irreversible binding to DNA that is thought to disrupt the normally routine DNA repair and cell division cycle, leading to controlled cell death, known as apoptosis.
Side-effects and the second-generation platinum drugs
Cisplatin is not a flawless medication. Early in clinical trials, side-effects quickly became apparent. The most major of these was the toxicity, which was particularly damaging to the kidneys, gastrointestinal tract and nervous system. The cause of these effects is the indiscriminate nature in which cisplatin, and other platinum-based cancer treatments, target all cells, healthy and tumorous. In addition, other problems associated with the administration meant that alternative cisplatin derivatives were sought.
The square-planar properties responsible for the directed DNA binding meant platinum metal complexes remained attractive. The first iteration of platinum drug development sought to discover a less toxic platinum-based analogue, considered the ‘holy grail’ of platinum metal complexes. The task was to find a complex either as active as cisplatin while eliminating the most serious side-effects, or that had increased potency – a drug’s effectiveness – allowing for a lower dose administration, effectively reducing the severity of the side-effects.
Thus began the renaissance of inorganic chemistry and decades of academic-industrial collaborations where Rosenberg sought further assistance of leading companies in the field of metallic chemistry. Working together with companies such as Johnson Matthey and the Institute of Cancer Research (ICR) meant that the second generation of platinum metal cancer drugs were synthesised and screened. Multiple analogues of cisplatin were tested, whereby the ligands were altered and tested. The most successful of these is known as carboplatin, which gained FDA approval in 1989. Compared with cisplatin, carboplatin offered a dramatic reduction in toxicity, but at a reduced activity. So important was the industrial collaboration that in 1991 Johnson Matthey, the ICR and the Royal Marsden Hospital were joint winners of the Queen’s Award for Technology for their discovery of carboplatin.
There are a few other second-generation drugs that have been approved for patient use; for example, oxaliplatin approved by the FDA in 2004. However, it is an inconvenient part of drug development, and research in general, that most platinum complexes screened ultimately end up pushed to the side-lines.
Resistance and the third generation of platinum drugs
Following the development of carboplatin and its commercial availability, researchers identified a new target. After years of research into new complexes that were effective replacements for cisplatin, attention shifted to types of cancer that weren’t so responsive to cisplatin. Compounding this issue was the fact that certain cancers that had previously been effectively treated by cisplatin had developed cisplatin-resistance and the tumours had recurred. The search now began to identify a new platinum complex that could treat such cancers. Thus, began the third generation of cisplatin drugs.
There are three major mechanisms by which a cell can be resistant to cisplatin.4 First, the mechanism of cisplatin influx – the mode of entry into a cell – can be prevented or slowed, inhibiting cisplatin’s entry into the cell. Alternatively, the mechanisms of cisplatin efflux – the modes of cell exit – are accelerated, removing cisplatin before it can interact with a cell’s DNA. Finally, the cancer cells themselves can evolve to improve the methods of DNA repair, meaning damage caused by cisplatin is repaired and cell apoptosis is not triggered. Owing to the similar mechanism of DNA binding between cisplatin and the second-generation platinum metal complexes, cell lines resistant to cisplatin were also resistant to drugs such as carboplatin. Thus, a platinum-based complex was sought with a different structure that would be active in cisplatin-resistant cells.
Two such platinum drugs that showed activity in cisplatin-resistant cells are known as satraplatin and picoplatin. Satraplatin was originally developed as an orally administered drug, a more desirable method of administration than the intravenous injection necessary for cisplatin and carboplatin. More importantly, during clinical trials satraplatin was found to remain active in cells that had developed cisplatin-resistance. Ultimately, satraplatin never made it to market, with the FDA announcing it had put its approval process on hold back in 2007.
Picoplatin was the final platinum metal complex to emerge from the Johnson Matthey–ICR collaboration. It has also shown activity in cisplatin-resistant cancers owing to the steric bulk introduced by an alternative nitrogen-based ligand. However, despite promising clinical trials, the drug never became commercially available.
The new renaissance of pharmaceutical research
Platinum metal complexes were revolutionary innovations for an era when cancer research was in its early days. Cisplatin and its metal complex derivatives are still essential therapies to this day, being offered to patients around the world supplied by the leading experts in fine chemicals industry including Johnson Matthey and Umicore. More than 50 years have now passed since Rosenberg’s original research detailing the effects of cisplatin on bacterial growth. Throughout this time, the scientific community’s collective technological and biological understanding has led the pharmaceutical industry to the precipice of a new renaissance of anti-cancer treatments.
Other therapies will eventually surpass cisplatin as a treatment for cancer, predominantly because of their targeting capabilities. Severe side-effects have pushed cancer treatments towards more targeted therapies that show reduced side-effects, owing to their increased specificity towards cancer cells. Innovative treatments such as antibody drug conjugates that deliver a cytotoxic treatment directly to a targeted cell and light-focused treatments involving bursts of directed lasers are some of the potential next-generation anti-cancer therapies. By offering reduced side-effects the patient is the beneficiary, undergoing a more comfortable treatment experience. Shifting away from indiscriminately cytotoxic small-molecule treatments will potentially relegate cisplatin and its analogues to relics from the past. But the scientific community should never forget the impact this one simple metal complex has had on modern medicine.
References
1 B Rosenberg, L Van Camp, T Krigas,Nature, 1965, 205, 698 (DOI: 10.1038/205698a0)
2 B Rosenberg et al,Nature, 1969, 222, 385 (DOI: 10.1038/222385a0)
3 L H Einhorn,J. Clin. Oncol., 1990, 11, 1777 (DOI: 10.1200/JCO.1990.8.11.1777)
4 L Kelland,Nat. Rev. Cancer, 2007, 7, 573 (DOI: 10.1038/nrc2167)
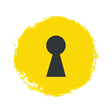
No comments yet