A sulfur iodide crystal discovered by US researchers could help solve some of the key problems of next generation lithium batteries. The material is 11 orders of magnitude more electronically conductive than elemental sulfur, drastically reducing the internal resistance in lithium-sulfur batteries. It also has a much lower melting point, which allowed electrode defects from repeated cycling to be healed by gently heating them. This allowed the battery to retain good capacity over 400 charge–discharge cycles.
Many researchers regard lithium-sulfur batteries as a key prospective advance in lithium battery technology because they could hold much more energy than today’s lithium-ion batteries. These usually use lithium cobalt oxide cathodes: during charging lithium deintercalates from the lithium cobalt oxide, oxidising the cobalt(III) to cobalt(IV). During discharge, this process is reversed, releasing electrons into the external circuit. Lithium-sulfur cathodes, however, work by electrochemical conversion. ‘Lithium reacts with sulfur to form lithium sulfide – that gives you the energy to form your lithium-ion battery,’ explains computational materials scientist Shyue Ping Ong at the University of California, San Diego. This means more lithium ions can be stored and more electrons released, as well as obviating the need for cobalt, which is plagued by supply-chain concerns.
However, elemental sulfur is highly insulating, which creates internal resistance. Moreover, the conversion is chemically complex and problematic in liquid electrolytes. ‘Even though the two end-members are solid and are not soluble in the electrolytes, the intermediates unfortunately are,’ explains experimentalist Ping Liu, also at UC, San Diego. ‘This helps to deal with the perfectly insulating nature of the elemental sulfur, but when you’re going back and forth from a solid to a liquid to a solid it’s very difficult to get reversibility.’ Solid state batteries, which naturally have inert electrolytes, help solve the second problem, but they exacerbate the first. Maintaining a conformal interface between solid sulfur and a solid electrode over repeated cycles is also challenging.
Liu and colleagues produced sulfur iodide molecular crystals by heating sulfur and iodine powder together. At the optimum stoichiometry of S9.3I, analysis by Ong’s group showed periodic I2 substitutions into the octahedral structure of elemental sulfur. The iodine added additional electronic energy levels, increasing the material’s conductivity by 11 orders of magnitude. Moreover, it disrupted van der Waals forces, lowering the melting point to 65°C.
The researchers tested their material in a solid-state battery, initially melting it into the electrolyte to achieve conformal interfaces. They found that, as a result of its greater electronic conductivity, it showed much higher capacity than a battery with a sulfur cathode. After 50 cycles, this had decayed slightly as defects had developed in the cathode interface. However, reheating the battery to 100°C restored its function by healing these defects, and with periodic reheating the battery retained 87% of its capacity after 400 cycles. The researchers are now working towards commercialisation of their technology. ‘The thermal management aspect is surprisingly easy to implement,’ says Liu. ‘Most of the challenge with today’s lithium-ion batteries with liquid electrolytes is that they need to be cooled.’
‘As an inorganic chemist and electrochemist who works on lithium-sulfur batteries, I’m very intrigued,’ says Linda Nazar of the University of Waterloo in Canada. She notes that the existence and nature of sulfur iodides was debated for over 100 years, with many chemistry textbooks saying no such compound was possible despite a report of one with a melting point of 65°C in 1908. She notes, however, that the capacity per unit electrode area of the cathode over 400 cycles is around 1mAh/cm2 – less than commercial lithium batteries today. In the extended data, however, the researchers show batteries that have 4–4.5mAh/cm2 capacity sustained over a ‘very promising’ 50 cycles. Extending this to hundreds of cycles ‘would constitute a big step change,’ she says.
Update: On 13 March 2024 the passage on battery charging/discharging was corrected.
References
J Zhou et al, Nature, 2024, DOI: 10.1038/s41586-024-07101-z
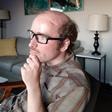
No comments yet