No morphological differences between living and non-living systems are yet known
In August 1996, US president Bill Clinton made a portentous announcement from the White House. He was talking about a rock.
‘Today, rock 84001 speaks to us across all those billions of years and millions of miles’, he said. ‘It speaks of the possibility of life. If this discovery is confirmed, it will surely be one of the most stunning insights into our universe that science has ever uncovered.’
The Martian meteorite ALH84001, picked up at Allan Hills in Antarctica in 1984, was then in the process of acquiring notoriety for revealing the Martians that never were. Researchers at NASA claimed in 1996 that inspection of the meteorite under the electron microscope revealed weird, wormlike nodules which could be ‘fossil remains of a past martian biota’.1
In fact, ALH84001 had a different message for us: about the danger of inferring life from morphology. It has never become clear what processes created the peculiar microstructures of the meteorite, but there’s no strong reason to believe they involved Martian microbes, and plenty of evidence that non-living processes can generate comparable shapes and forms. Perhaps chief among these are reaction-diffusion systems, of which the periodic precipitation process discovered in 1896 by German chemist Raphael Eduard Liesegang may be a variant.2 It’s now widely believed that some of the oldest putative microfossils on Earth,3 showing filamentary structures once thought to be fossil bacteria, are also in fact inorganic.
Perhaps a little chastened by the damp squib of ALH84001, NASA scientists began in the late 1990s to develop an artificial-intelligence system that would be able to distinguish the living from the non-living on the basis of morphology. They called it the D’Arcy Machine, after Scottish zoologist D’Arcy Wentworth Thompson, whose epic 1917 book On Growth and Form was one of the most comprehensive early efforts to understand how complex morphologies arise in both living and abiotic systems.
The project was led by chemist David Noever of NASA’s Marshall Space Flight Center in Alabama. The idea was that a neural network would be trained on all known biological forms on Earth to find features diagnostic of life. ‘By repeatedly comparing and contrasting learnable imagery’, NASA claimed, ‘a D’Arcy machine would… give us an interplanetary version of D’Arcy Thompson’s classic [book].’
It seems that nothing notable came from the project. But might that be because there isn’t really any reliable morphological distinction between the living and abiotic?
That question was raised in a recent meeting in Tokyo, Japan, titled ‘Diversity of Biological Patterns and Forms in Nature’ and hosted by the Meiji Institute for Advanced Study of Mathematical Sciences. While most of the presentations discussed the dizzying array of forms that the living world produces – few are more astonishing than those of South American treehoppers – chemist Oliver Steinbock of Florida State University offered a reminder of how shape alone is a poor guide.
In the chemical garden
Steinbock described the complex and often beautiful forms of chemical gardens, made by precipitating salts within a solution of sodium silicate (water glass). The repeated formation of soft inorganic membranes followed by their breaching due to osmotic pressure gives rise to twisting, bulbous mineral columns. Isaac Newton studied such structures in the 17th century and proclaimed that they had something ‘vegetative’ about them.
Shape is one thing – but even the kind of complex motion we might intuitively associate with life doesn’t necessarily provide clinching evidence. Steinbock showed that short lengths of the tubular structures in manganese chemical gardens can act as self-propelled particles – active matter – in solutions of hydrogen peroxide, catalytically generating oxygen that bubbles out of the tube end and propels it forward.4 The trajectories of these particles can be surprisingly complicated, especially when several interact hydrodynamically with one another. In particular, the particles, typically a few millimetres long, can appear to congregate like microbes into a ring pattern – driven by the surface tension and buoyancy of a collective oxygen bubble in the ring’s centre.5
These little tubules can also show periodic cycles of ascent and descent in the liquid due to the buoyancy of an oxygen bubble and its release at the surface.6 It’s tempting to read this motion visually as some kind of purposeful behaviour. The fact is that we seem naturally inclined to infer life and purpose in all manner of complexity: we are prone to what you might call ‘biotic pareidolia’, the analogue of our impulse to see faces in non-living structures (including the rocks of Mars!).
Can today’s immensely powerful deep-learning AI, potentially free from such cognitive biases, do better in identifying what is truly life-like? Given recent developments that have exposed how strongly we project life and thought onto AI itself, I wonder about that. If AI can persuade us that it’s alive, it might not have much trouble convincing us that something else is.
References
1 D S McKay et al., Science, 1996, 273, 924 (DOI: 10.1126/science.273.5277.924)
2 H Nabika, M Itatani and I Lagzi, Langmuir, 2020, 36, 481 (DOI: 10.1021/acs.langmuir.9b03018)
3 W Schopf (ed.), Earth’s Earliest Biosphere. Princeton University Press, Princeton, 1991.
4 Q Wang, P Knoll and O Steinbock, J. Phys. Chem. B, 2021, 125, 13908 (DOI: 10.1021/acs.jpcb.1c09088)
5 P Kumar et al., Soft Matter, 2022, 18, 4389 (DOI: 10.1039/D2SM00395C)
6 Q Wang and O Steinbock, Soft Matter, 2023, 19, 2138 (DOI: 10.1039/D2SM01681H)
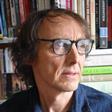
No comments yet