Nina Notman speaks to the researchers exploring medical applications for silkworm silk
Cultivating silk moths to produce silk threads for weaving into fabric is thought to have begun in China around 5000 years ago. Artificial selection over thousands of moth generations produced Bombyx mori, the domesticated moth that feeds on mulberry leaves and is still used to produce silk today. There is a multibillion-dollar global textile market for silk, but it also has some lesser known non-textile applications – including as surgical sutures.
Silk sutures have been in use since around 600BCE, and they are still used today following certain types of surgery. Around 30 years ago, David Kaplan, a biomedical engineering professor at Tufts University in Massachusetts, US, had a lightbulb moment, when he realised that silk could be useful for other medical applications too. This led to the birth of a new research field, which has resulted in a handful of approved products worldwide – so far. Many more silk medical products are in clinical trials and preclinical testing.
The cocoon of the Bombyx mori silkworm contains around 70% fibroin; a fibrous protein that is inherently biocompatible and has a tensile strength similar to steel. Fibroin also has a unique combination of other attributes that make it particularly appealing for medical applications. There are decades of evidence that silk sutures can be used safely in the body. There is also a robust supply chain manufacturing Bombyx mori silk under controlled conditions for a reasonable price. If a biotechnologist was asked to produce a high-performance protein with all the positive attributes of silk, ‘they’d laugh and say: I can do it for £10,000/kg’, says Chris Holland, a materials science and engineering lecturer at the University of Sheffield, UK.
A natural solution
But silk’s most exciting characteristic is its ability to be reformulated into a multitude of different forms. ‘It is possible to chemically unspin silk and then reformat it to use as a building block to create a whole range of different types of materials,’ says Holland. These new forms include porous scaffolds, hydrogels, films, nano- and microparticles and dense plastic-like materials. This opens the door to a huge range of potential applications.
Fibroin is a block co-polymer comprised of very long, highly hydrophobic chains joined together by short hydrophilic linkers. The protein is soluble in water inside the silkworm’s silk gland. But as it leaves, it is forced through a cylindrical nozzle, which triggers it to self-assemble into a solid insoluble fibre. ‘Silk is spun via a controlled protein denaturation,’ says Holland. ‘It’s the equivalent of cooking an egg, but instead of using heat, you are using mechanical flow to cook the silk proteins and transform them from a hydrated state to a dehydrated and aggregated state.’
When spun, fibroin is arranged as β sheets, a highly ordered protein secondary structure type with hydrogen bonds holding the protein chains together. To create alternative forms of silk, the β sheets first have to be physically disassembled. There are a number of ways to do this. ‘In our lab, we use lithium chloride salts to deconstruct the hydrogen bonding that holds the fibroin protein together, and that converts the solid silk fibre material in the cocoon into a water solution. Next, we dialyse off the lithium salt to leave the protein in water,’ says Kaplan.
‘Then we can induce a new confirmation,’ adds Antonella Motta, an industrial bioengineering professor at the University of Trento in Italy. This can involve reforming the β sheets with more robust crosslinkers or adding in some alternative secondary structure types such as α helixes. The α helix structure is spring-like and adds some elasticity to the material, Motta explains. Changing the temperature, solvent, pH or manipulating mechanical stresses during re-assembly are all ways to control the form in which fibroin reassembles. Another common approach is to chop the polymer’s long chain into smaller peptide pieces, which prevents it assembling into as ordered a structure as before. Better understanding how nature spins its silk can support research into how it can be formed differently in the lab (see Learning from nature below).
Learning from nature
Chris Holland at the University of Sheffield is working to better understand how the Bombyx mori silkworm spins its silk. He hopes that by unlocking the secrets of the natural process, it will be possible to improve the way silk can be respun and reformulated in the lab. The natural process is too much of a ‘black box’ at the moment, Holland explains.
‘We are interested in understanding how the silk transforms from a stored liquid gel [in the silk gland] into a final solid fibre,’ Holland says. Parameters he explores include the speed at which silk is spun, how and when it’s physically manipulated by the animal during the spinning process, and the role of pH and calcium and potassium ion concentration changes. He also takes unspun silk – from the Bombyx mori silk gland – and recreates the conditions they have observed in the lab, using rheometers and other analytical techniques to probe how the silk structure develops during the spinning process.
Controlling chemistry
Chemistry can also be used to control fibroin’s reassembly process and therefore its final form and properties. Most commonly, this means adding chemical cross linkages. Established techniques for doing so include using enzymes to create di-tyrosine bonds between the tyrosine amino acids in silk’s polymer backbone. This type of crosslink adds elasticity to the silk and is therefore popular for creating silk hydrogels.
Adding new side chains – with different functionalities – to the polymer backbone is a less used tool for changing silk’s properties. It can be challenging, as fibroin’s backbone is primarily comprised of the non-reactive amino acids glycine and alanine. One way around this is to genetically engineer silkworms so that the silk they produce contains unnatural, reactive amino acids onto which side chains can be added. Hidetoshi Teramoto, a biotechnology researcher at the National Agriculture and Food Research Organization in Ibaraki, Japan, is one of the scientists pioneering this approach. He is able to incorporate an unnatural amino acid with an azide group into fibroin. This allows side chains to be added using the classic click chemistry azide–alkyne cycloaddition. ‘When we incorporate that azide-bearing amino acid into silk, the silk can be clicked with virtually any kind of molecule with an alkyne,’ says Teramoto. Antibacterial silk is one application being explored for his functionalised silk.
But genetic engineering isn’t always needed. Kaplan’s lab has developed a chemistry-based approach for attaching side chains to the polymer backbone of natural silk. Perfluorocarbon side chains can be attached transforming the protein’s naturally hydrophilic surface into one that is more hydrophobic than Teflon. This takes multiple steps. The hydroxyl groups on several of fibroin’s minor amino acids (including serine) are first turned into carboxylic acids, and primary amine groups are then attached to these before fluorocarbon chains are attached to the amines. Each step of this chemical synthesis is performed under mild conditions. Applications Kaplan foresees for this fluorinated silk includes medical devices that resist damage from water and other biological materials and therefore last longer.
Restoring voices
In recent years, fibroin-based medical products have seen approval for uses including therapeutic silk clothing for treating chronic skin conditions and silk-based wound dressings. The first silk product approved by the US Food and Drug Administration (FDA) for use inside the body was the now discontinued SERI Surgical Scaffold. This porous silk scaffold originated in Kaplan’s academic labs and reinforced soft tissue after plastic and reconstruction surgery. It hit the market in 2013, before being withdrawn in 2021.
In 2018, a second silk-based tissue engineering scaffold, which also started life in Kaplan’s labs, gained FDA clearance. Silk Voice was cleared for the treatment of damaged vocal cords and was brought to market by Sofregen Medical, a spin out company based in Massachusetts. The product is a bulking agent able to restore the voice immediately after application. It also acts as a scaffold to support long-term regeneration of the damaged collagen soft tissue in the vocal cords. The scaffold takes 18–24 months to degrade, ‘providing a substrate to facilitate regeneration of the damaged tissue’, explains Joseph Brown, Sofregen Medical’s chief technology and operations officer.
Silk Voice is comprised of very soft, porous microparticle sponges which exhibit shape memory properties. ‘If you take a cross section of one of these porous particles, it’s mostly void space … that tissue and cells can infiltrate deep into,’ says Brown. ‘Our sponges have the ability to be compressed or stretched and maintain their shape after those external forces have been alleviated.’ This elasticity is important in vocal cords because they oscillate so much.
The silk nanoparticles stick together after they have been injected into the vocal cords, which allows them to be delivered minimally invasively. The length of the fibroin chains is used to control the final structure formed. ’Once delivered, the particles cohere together to volumise and provide mechanical bulking to the tissue,’ Brown says. Sofregen Medical is currently testing injectable silk dermal fillers in the clinic, which provide volumetric bulking while collagen grows into its place. The goal is to achieve a long-lasting correction, says Brown.
Kaplan is not the only academic working on exploiting silk’s potential for tissue engineering applications. Many research groups around the world share this goal with silk having also been shown to support the growth of bone, cartilage and skin. In the EU, Motta is part of a Horizon 2020 consortium project exploring silk and other novel materials for tissue engineering. ‘We are focused on [developing] sustainable materials for biomedical applications, particularly bone and skin,’ Motta explains.
Rethinking vaccines
Tissue engineering is just the tip of the iceberg when it comes to silk’s potential for medical applications. Vaxess Technologies in Massachusetts, for example, is using silk microneedles in sustained-release vaccine delivery patches. This technology was also spun out of Kaplan’s academic labs. The Mimix patches recently completed Phase 1 trials against influenza and are also being explored for the delivery of mRNA vaccines, including Covid-19, and therapeutics. The Phase 1 trial found that slowing down the release of antigens into the body may decrease the frequency with which typical influenza vaccine side effects such as fatigue and headaches occur. ‘There were very few adverse effects from our vaccination compared to traditional vaccines delivered by needle and syringe,’ says Jonathan Kluge, vice-president of research and development at Vaxess Technologies.
The Mimix patch has an array of microneedles whose tips contain a combination of silk and active ingredient. The needle shafts are water-soluble and dissolve in less than five minutes when in contact with the skin’s interstitial fluid. Once the patch is removed, the tips remain under the skin. ‘Those tips are now embedded [under the skin] and break down releasing the cargo by diffusion over time,’ Kluge says. It takes days to months for the entire payload to be released. The breakdown rate depends on the secondary structure of the silk, which the company can control by the length of the fibroin chains.
The patches are stable at room temperature making them easier to distribute than traditional vaccines, explains Kluge. ‘The improved shelf stability that comes from the dried format and the stabilisation features of fibrin that protect the vaccine cargo,’ he adds. The end goal is a self-administered product than can be used in non-medical settings, including in the home.
There were very few adverse effects from our vaccination
Silk’s potential as a tool to protect drugs and control their delivery in the body is also being explored by a number of academic groups. Philipp Seib, a pharmaceutical technology professor at Friedrich Schiller University Jena in Germany, is creating silk hydrogels and nanoparticles for this purpose.
Seib’s silk hydrogels protect drug molecules during direct injection into a defined target location. Injecting these into the brain to treat stroke damage is one application his group is exploring, and the hydrogels have entered pre-clinical trials. ‘Ours and other groups have injected these [types of] systems in animal models and seen positive responses,’ Seib says.
The silk nanoparticles, meanwhile, are for intravenous injections. Seib is designing these to protect the drug and to control their destination in the body. ‘Because we’re encapsulating the drug in a nanoparticle, we are restricting where it can go,’ he says. The nanoparticles circulate in the blood until they get to a location where blood vessels are leaky, such as those in solid tumours. The lysosomal enzymes and low pH in these areas trigger the disintegration of the nanoparticle to deliver its anti-cancer drug cargo. As well as delivering the drug exactly where it is needed, this also reduces side effects caused by its unintentional delivery elsewhere.
Both the drug-carrying silk hydrogels and nanoparticles are held together by physical crosslinkages. Seib is also working on hydrogels with di-tyrosine chemical crosslinkages for delivering cell therapies.
Going natural
The vast majority of silk textiles are produced by the silkworm of the Bombyx mori moth, which was domesticated thousands of years ago. The same breed is also being exploited for medical applications by most researchers – but not quite all. Biman Mandal, a bioengineering professor at the Indian Institute of Technology Guwahati, India, is an outlier who is using wild silk instead.
The wild silks that Mandal uses include those produced by the Antheraea assama and the Antheraea mylitta moths. These have a subtly different polymer backbone to Bombyx mori silk. Specifically, the protein has arginine–glycine–aspartate sequences in its backbone, which Mandal says is desirable for tissue engineering applications. This sequence helps with cell binding and cell attachment without the need for chemical modifications, he explains. Another approach unique to his lab is extracting the liquid silk proteins from the silk gland before it is spun. This allows him to skip the unspinning step when manufacturing a silk hydrogel or another novel form of silk. ‘This [also] gives our silk very unique biophysical and mechanical properties.’ he explains.
Mandal has explored a variety of tissue engineering applications for his wild silk products, including supporting the growth of skin, bone, and other tissues, as well as blood vessels.
Resisting adhesion
Another potential application being explored for silk hydrogels is the prevention of painful adhesions after orthopaedic surgeries. Xiaoqin Wang, an engineering professor at Soochow University in China, has developed biodegradable silk–polyethylene glycol (PEG) hydrogels to support wound healing and prevent adhesion formation between scar tissue and bone.
This product is currently in clinical trials in China. Like silk, PEG is already long approved for use inside the body. Wang’s silk–PEG hydrogels have enhanced mechanical properties and stability compared to pure silk hydrogels, he explains. The hydrogels form when a silk solution is mixed with a solution of low molecular weight PEG. The temperature of the gelation process controls the fibroins secondary structure. ‘We only use physical crosslinking, no chemical crosslinkers,’ Wang explains. He is also developing an injectable hydrogel that forms inside the body.
In the coming years, the number of approved medical applications for silkworm silk is expected to grow exponentially due to the material’s unique combination of useful characteristics and – especially – its versatility. The vast majority of this silk – but not all (see Going natural above) – will be manufactured by the Bombyx mori silkworm that was domesticated by our ancestors thousands of years ago and already available in very large supplies. Scientists and engineers do not yet have the tools to manufacture a synthetic polymer that is anywhere near as good or as cost effective as this one produced by nature. ’If you gave the design specs [of silk] to a chemical engineer and asked them to reproduce them, I think their heads probably pop,’ says Holland.
Nina Notman is a science writer based in Salisbury, UK
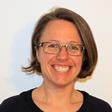
No comments yet