Are water microdroplets chemical dynamos or are their apparent effects experimental artefacts? Rebecca Trager investigates
When a team led by Stanford University’s Richard Zare announced in 2019 that sprays of microscopic water droplets spontaneously produce hydrogen peroxide it attracted both excitement and scepticism. These days Zare is doubling down, arguing that many applications for catalysis – including nitrogen fixing, converting methane to methanol and transforming carbon dioxide into valuable chemicals – can be addressed with water microdroplets.
‘It’s going to make it possible to do chemistry in a much greener, [more] ecofriendly manner,’ says Zare, who pioneered using lasers to study chemical reactions and developed laser-induced fluorescence spectroscopy more than 50 years ago. Now, almost all his research is focused on water microdroplets. Not everyone believes water droplets are highly reactive, but Zare says ‘the evidence is overwhelming.’
Graham Cooks, an analytical chemist at Purdue University in the US with expertise in mass spectrometry, wasn’t surprised by Zare’s team’s findings. It was already well-established that unusually fast chemistry happens in tiny drops of water, notes Cooks, who was part of a team that first reported accelerated reactions in sprayed microdroplets over a decade ago.1 His work showed water microdroplets accelerated chemical reactions by a factor of up to a million. Zare suggests that those rates can be even higher.
But Christian George, a chemist with the National Centre for Scientific Research in France whose research focuses on atmospheric aerosols, says that acceleration has yet to be proven. ‘There is lots of debate about this acceleration of chemical reactions in microdroplets,’ he states. ‘We were not able to reproduce [Zare’s team’s] experiments,’ he adds, noting that his group never published on this and is currently busy with other things.
How it started
Zare’s team made its hydrogen peroxide discovery while trying to synthesise gold nanostructures in microdroplets,2 armed with the knowledge that Michael Faraday had made gold films with lemon juice. They added sodium borohydride – which Zare calls ‘modern lemon juice’ – to water microdroplets and found that the reaction occurred 100,000 times faster than in bulk liquid. As a control experiment, the team removed the reducing agent – the sodium borohydride – yet still produced gold nanoparticles. ‘That was a shock to us,’ says Zare.
The team searched for the cause of this reduction and where the electrons came from, and ultimately recorded that sprayed water microdroplets spontaneously produce around 30µM hydrogen peroxide. This immediately grabbed the attention of Himanshu Mishra, an environmental science and engineering professor at King Abdullah University of Science and Technology (Kaust) in Saudi Arabia.
Mishra asked his students to repeat the Stanford experiment, but they couldn’t. Then, the team used an ultrasonic humidifier like the one Zare’s group used and got a signal of 1μM hydrogen peroxide. Mishra originally theorised that this was due to evaporation or to shockwaves in the system.
There is lots of debate about this acceleration of chemical reactions in microdroplets
Christian George, CNRS
A follow-up study by Zare’s team in 2020 found that water vapour condensing in air to form microdroplets on cold surfaces contained hydrogen peroxide in concentrations of around 68µM – about double the amount they found in sprayed droplets.3 They suggested the cause was a large, intrinsic electric field at the air–water interface. This result rocked Mishra. ‘It contradicted our two previous hypotheses because when you are condensing water there are neither shockwaves nor evaporation,’ he says. But then Mishra came across warnings about possible ozone contamination when trying to detect hydrogen peroxide, and a eureka moment followed. ‘I jumped from my sofa, and I ran to the lab,’ he recalls. ‘We have ozone generators, so we made a little spray and we exposed it to ozone, and for the first time in a year of hitting our heads against the wall, that strip that is sensitive for hydrogen peroxide turned blue.’
Zoning in on ozone
Working in a glove box, the Kaust researchers concluded that water microdroplets – sprays or condensates of any size – can’t produce hydrogen peroxide without ozone.4 They suggested the hydrogen peroxide was caused by tiny microbubbles formed by cavitation when an ultrasonic humidifier vibrates the water at a megahertz frequency. When these bubbles burst there are very high temperatures and pressures that form OH radicals, they thought.
I have a lot of trouble getting people to believe what I’m saying
Richard Zare, Stanford University
Since the Kaust study was published in 2022, Zare and his colleagues have conducted clean experiments that eliminated ozone from their system. In the resultant paper, published in April 2023,5 the team recorded hydrogen peroxide concentrations ranging from 0.3μM to 1.5μM, depending on flow conditions – a significant decrease from their initial findings.
‘The Kaust people claimed that it all came from ozone,’ Zare says. ‘We have scrupulously removed ozone by using highly purified nitrogen – sending it through an ozone scrubber and confirming with an ozone meter that there was no ozone, and we still saw hydrogen peroxide,’ he continues. ‘And we know we see it because we can use nuclear magnetic resonance to identify it.’
But Mishra underscores that without ozone, the hydrogen peroxide signals that Zare detected have decreased by two orders of magnitude. ‘There is no controversy – it is very clear that the majority of the effect that they claimed was an artefact – there is no doubt about that,’ he says.
However, Zare points to an international team including George that published a paper in April 2023 concluding that it is ‘highly unlikely’ the observed interfacial OH radical formation in their experimental systems is related to ozone contamination.6 They also determined that it is not related to any impurity of the chemicals used, or any ‘uncareful’ experimental procedures.
The disagreement among experimentalists in this area centres around the fact that Zare’s group ‘had to revise their originally reported hydrogen peroxide concentrations down,’ explains Teresa Head-Gordon, a computational and theoretical chemist at the University of California, Berkeley. ‘However, two independent experimental groups found concentrations of hydrogen peroxide above the background and detection limit, and therefore something is going on,’ she adds. ‘So let’s find out what it is.’
Chemistry at interfaces
‘I fully recognise that there would be some suspicion about all of this – it’s counterintuitive in some sense,’ acknowledges George, who is also the deputy director of the Research Institute on Catalysis and the Environment in Lyon. ‘But … electrified interfaces are a key topic in many aspects of surface science, so why not naturally on the air–water interface where an electric field was discussed as being present for decades to centuries.’
Indeed, what makes water microdroplets so special, Zare says, is that charge separation when positive and negative ions adsorb differently to the air–water interface creates a powerful electric field. This electric field, when combined with the partial solvation of such ions at the interface – where three-dimensional solvation shells are not possible – makes the interface very reactive. Zare’s team has collaborated with researchers at Columbia University in the US to measure that electric field using Stark spectroscopy.7 They found it’s about 107 volts per centimetre.
‘You cannot make an electric field that strong in the laboratory between two parallel plate capacitors without having lightning occur long before you try to reach that limit,’ Zare notes. Computational work by Head-Gordon and colleagues supports his electric field hypothesis.8
The electric fields in water microdroplets can drive chemical reactions that don’t occur in bulk water, says Zare. All the action appears to occur on the exterior of the water droplet, and there are several effects at play. First, he says three-dimensional hydrogen bonding no longer occurs on this surface and so the molecules are partially solvated, meaning they are much more reactive. Second, many more ions are being formed and that means the droplet’s surface becomes more aligned and ordered at the interface.
‘I have a lot of trouble getting people to believe what I’m saying, but I’m telling you what’s going on,’ Zare continues. ‘Water itself falls apart into H+ and OH–, and at the interface an electron jumps from the OH– to the H+ to form the radicals H・ and HO・,’ both of which are highly reactive.
Mishra’s team, however, has an alternative explanation. ‘We have figured out where that damned thing is coming from,’ he says. They claim – in a preprint published on the arXiv server in November 2023 – that hydrogen peroxide forms when microdroplets meet a solid surface.9 ‘Our findings bust several myths surrounding the spontaneous formation of H2 O2 at the air–water interface, including the instantaneous ultrahigh electric fields, the “micro” scale of droplets and empirical arguments based on hydration enthalpies,’ the preprint concludes.
Mixed feelings
While Head-Gordon tends to see the glass is half full because hydrogen peroxide has been detected above a background even without ozone, others like Ryan Sullivan of Carnegie Mellon University in the US have doubts. ‘Many physical atmosphere chemists who I regard as very careful experimentalists that do a lot of work on aerosol chemistry and interfacial chemistry do not think that this idea that oxidants are spontaneously being produced in microdroplets is real – they are all quite convinced it’s contamination or some sort of artefact,’ states Sullivan, who has more than two decades of expertise in the chemistry of atmospheric aerosol particles.
‘They point out, and this is also my leaning now, that because so many of our experiments over the last several decades in atmospheric chemistry involve aerosols that are often even smaller than what Zare is working with – often they are submicron – so if droplet accelerated chemistry is explaining the spontaneous production of oxidants then it should happen even more in smaller droplets because you have more surface-area-to-volume ratio,’ Sullivan states. Some of these sceptics have done their own experiments but haven’t necessarily published them, in part because it can be hard to publish negative results, he says.
We have figured out where that damned thing is coming from
Himanshu Mishra, King Abdullah University of Science and Technology
In fact, Mishra recalls that during the work that led to his group’s ozone finding, the large quantities of negative results frustrated his graduate students and some repeatedly requested to switch projects. Nevertheless, he says, they persevered for over two years in the face of innumerable null results.
Other experts like Agustin Colussi, a physical chemist who has worked on interfacial chemistry and electrochemistry at Caltech for more than two decades, argue that producing hydrogen peroxide from water is endothermic, so an isolated water droplet cannot produce a higher energy product. ‘You need some external energy input,’ he says. ‘Internal electric fields by themselves cannot produce hydrogen peroxide because this is against thermodynamics – internal rearrangements won’t help you.’
Meanwhile, some in the field, like Head-Gordon, suggest this thermodynamic argument is incomplete because it describes bulk water thermodynamics, not what happens at the microdroplet interface. Research has shown that it is much easier to liberate an OH radical at this interface. ‘We have done very high quality, unambiguous calculations showing that if I’m a hydroxide ion at the air–water interface, the ability to liberate an electron is much easier than if I were in the bulk,’ Head-Gordon says.
Motivated by applications
The debate surrounding water microdroplet chemistry is set to continue. Even so, Zare remains motivated by his belief that water microdroplets can instigate useful chemical reactions, if not enhance the reactivity and rate of traditional catalysis. Within the last nine months, he and his colleagues have published a significant amount of work in this area. This includes papers reporting that water microdroplets can help turn carbon dioxide and nitrogen into urea;10 nitrogen into ammonia;11 benzoic acid into phenol;12 and toluene into phenylacetic acid.13
‘Normal water, you think puts out fire and is supposedly benign and inert, but I’m telling you water droplets at their surface are really chemical dynamos,’ Zare concludes.
References
1 M Girod et al,Chem. Sci., 2011, 2, 501 (DOI: 10.1039/c0sc00416b)
2 J K Lee et al,Nat. Commun., 2018, 9, 1562 (DOI: 10.1038/s41467-018-04023-z)
3 J K Lee et al,Proc. Natl. Acad. Sci. USA, 2020, 117, 30934 (DOI: 10.1073/pnas.2020158117)
4 A Gallo Jr et al, Chem. Sci., 2022, 13, 2574 (DOI: 10.1039/d1sc06465g)
5 M A Mehrgardi, M Mofidfar and R N Zare, J. Am. Chem. Soc., 2022, 144, 7606 (DOI: 10.1021/jacs.2c02890)
6 K Li et al,Proc. Natl. Acad. Sci. USA, 2023, 120, e2220228120 (DOI: 10.1073/pnas.2220228120)
7 H Xiong et al,J. Phys. Chem. Lett., 2020, 11, 17, 7423 (DOI: 10.1021/acs.jpclett.0c02061)
8 H Hao I Leven and T Head-Gordon,Nat. Commun., 2022, 13, 280 (DOI: 10.1038/s41467-021-27941-x)
9 M A Eatoo and H Mishra,arxiv, 2023, DOI: 10.48550/arXiv.2311.11133
10 X Song et al, J. Am. Chem. Soc., 2023, 145, 25910 (DOI: 10.1021/jacs.3c10784)
11 X Song, C Basheer and R N Zare, Proc. Natl. Acad. Sci. USA, 2023, 120, e2301206120 (DOI: 10.1073/pnas.2301206120)
12 Y Meng R N Zare and E Gnanamani, J. Am. Chem. Soc., 2023, 145, 35, 19202 (DOI: 10.1021/jacs.3c08638)
13 Y Meng, E Gnanamani and R N Zare, J. Am. Chem. Soc., 2023, 145, 14, 7724 (DOI: 10.1021/jacs.3c00773)
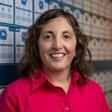
Water microdroplet chemistry is contentious, here’s why
- 1
Currently reading
Water microdroplet chemistry is contentious, here’s why
- 2
No comments yet