Four-atom molecules linked together by microwaves have been cooled to a record-breaking hundred-billionth of a degree above absolute zero.
Cooling matter to such frigid temperatures is exceptionally difficult as molecules consist of multiple moving atoms, so have more kinetic energy which in turn warms them up. Scientists are interested in cooling molecules to these ultracold temperatures as it enables the study of quantum dynamics and chemical reactions, with polyatomic molecules emerging as a platform for quantum information processing.
‘Supermolecules’ were first theorised two decades ago involving a novel type of weak binding between polar molecules in an electric field. However, it is only now that, for the first time, experimentalists at the Max Planck Institute of Quantum Optics in collaboration with the Chinese Academy of Sciences have directly observed the existence of such a ‘supermolecule’ – a ‘field-linked’ tetratomic molecule. This phenomenon occurs when a unique bound state forms as a result of electrical forces and, while it is weaker than typical chemical bonds, has bonds that are several hundred times longer. The complexity of these molecules, compared with diatomic molecules, however, poses challenges when employing conventional cooling techniques such as direct laser cooling and evaporative cooling. ‘We have to cool down the molecule to very low temperatures such that the thermal excitation cannot affect the bonds between these molecules and they can form a bound state,’ explains Tao Shi at the Chinese Academy of Sciences, adding that ultracold conditions are necessary for the formation of the molecules.
The team created the weakly bound ultracold (NaK)2 molecule using a new approach – the electroassociation of a pair of NaK molecules. Each NaK molecule is hit by a polarised microwave field which induces rotating dipole moments that can host stable tetratomic bound states. ‘[The] microwave field is like a magic scissor that can reshape the interaction between two different dipolar molecules, and also form a shield between two molecules, such that they cannot be very close to each other,’ says Shi. This microwave shielding allows for the formation of stable multi-atom molecules and without it evaporative cooling would lead to the two particles getting too close to each other and vanishing from the system. This method, termed ‘field linked resonance’ with control of the intensity of the microwave field, enabled the observation of (NaK)2 molecules using evaporative cooling. The researchers cooled the molecule to 134 nanokelvin – a record-low temperature for a tetratomic molecule. At a critical (low) temperature, novel quantum phases can be detected, and these molecules can be used for quantum computation and precision measurement. ’We know exactly the energy of this molecule such that…you shine this laser [and] you can see the shape of the complicated molecule,’ explains Shi.
‘The exciting thing about this is the generality – this works in principle for many polar molecules,’ says Simon Cornish, a physicist at Durham University. ‘Bringing the molecules into the ultracold regime gives the experimental control needed to explore many-body phases.’ Ultracold chemistry provides an opportunity to study the rich internal structure of molecules compared with atoms – ‘we can really realise chemical reactions that are forbidden at [room] temperature that can happen in this ultracold condition’, adds Shi.
References
XY Chen et al, Nature, 2024, 626, 283 (DOI: 10.1038/s41586-023-06986-6)
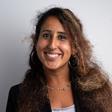
No comments yet