Using scanning tunnelling microscopy (STM), researchers have observed the piece-by-piece assembly of 2D dynamic covalent polymers – the basic building blocks of covalent organic frameworks (COFs). These detailed images will help understand complex growth mechanisms, potentially paving the way to tailored synthetic stacked structures.
‘The growth of two-dimensional COFs involves complicated chemical conversions, like the constant formation and breakage of bonds,’ explains Steven De Feyter, from KU Leuven in Belgium, who co-led the study. Moreover, other processes related to crystallisation also come into play. ‘However, little was known about the mechanistic and kinetic aspects of these reactions,’ he adds. Now, STM provides a proper picture of the polymerisation process, clearly showcasing monomers, oligomers, and their evolution over time.
‘The STM takes pictures every minute, which surprisingly coincides with the reaction rate, allowing a step-by-step monitoring of the whole process,’ comments co-leader Aurelio Mateo-Alonso at Polymat in San Sebastián, Spain. Initially, researchers observe the formation of small crystallisation nuclei, formed by single structures of pyrene-2,7-diboronic acid – a common monomer in the COF world. Dimers and small oligomers then appear and disappear, until their size is big enough to kick off the polymerisation process. ‘Such first images showcase the dynamic nature of nucleation and elongation in these materials,’ explains Mateo-Alonso. Additionally, researchers carried out computational simulations to complement the conclusions, and even developed new modelling methodologies.
Observing 2D-COFs under the microscope is technically challenging, according to nanomaterials expert Leslie Hamachi from the California Polytechnic State University, US. ‘Researchers have overcome the [challenges] and observed the growth of 2D polymers in real time, under ambient conditions, thus enabling fundamental insights on how these materials grow,’ she says. Understanding the crystallisation mechanisms could lead to reductions in defects and tailored orientations, both often desired in real-life applications. ‘As synthetic methods improve and the materials quality increases, [we could] see a dramatic increase in performance,’ explains Hamachi.
‘[This study] confirms our long-held belief for the COF formation mechanism,’ adds Omar Yaghi, materials expert and COF pioneer at the University of California Berkeley, US. The process behind the formation of 2D-COF layers had been ‘described and speculated’, he says, but ‘observing it is quite powerful’. The chemistry of these two-dimensional materials is increasingly interesting because of their unique properties. ‘2D COFs may [find applications] in designer membranes and even elements in electronics, their versatility … in terms of design and functionality [is] only rivalled by some metal–organic frameworks,’ adds Yaghi.
So far, the authors have only deciphered the fundamental parameters of 2D polymerisation – the conclusions don’t translate to three-dimensional COFs, as their formation follows different mechanisms. ‘[These] syntheses involve interlayer stacking processes and aren’t confined to on-[surface] growth,’ says Hamachi.
‘Given the influence that elucidating mechanisms had on the synthesis of [traditional] polymers, we envision these findings could enable the preparation of 2D COFs with similar levels of precision and tuneability,’ says Mateo-Alonso. These results could accelerate the preparation of other tailor-made structures featuring 2D COFs, such as heterostructures – ‘sandwiches’ of different layered materials, such as graphene and transition metal dichalcogenides. Heterostructures combine the best of both worlds and, among other things, they could lead to innovative applications in catalysis and electronics.
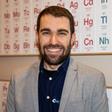
No comments yet