Oxygen-27 and oxygen-28 are the heaviest oxygen isotopes that have ever been created. However, initial experiments suggest that oxygen-28 does not possess the ‘doubly magic’ properties predicted that would confer unusual stability over other isotopes.
Studying isotopes with unusual neutron-to-proton ratios can provide researchers with unique insight into how atomic nuclei are structured. Of particular interest are nuclei that possess a ‘magic number’ of protons or neutrons, in which these nucleons are arranged into complete shells. Nuclei with closed shells of protons or neutrons tend to be particularly stable. A nucleus with a magic number of both protons and neutrons is known as doubly magic and such atoms are known to be unusually stable.
An international research team led by the Tokyo Institute of Technology’s Yosuke Kondo set out to investigate these phenomena using the world’s most powerful particle accelerator at the Riken Nishina Center in Japan. With 20 neutrons and eight protons, oxygen-28 would be the heaviest oxygen isotope ever created and would be expected to have doubly magic properties. The researchers were keen to see whether these characteristics would hold, given oxygen-28’s very high neutron-to-proton ratio.
The team made the isotope by bombarding an array of beryllium foils with calcium-48, causing the calcium nuclei to fragment. Fluorine-29 nuclei, which have the same number of neutrons as oxygen-28, were separated from the resulting products and then fired at a liquid hydrogen target. This collision caused some of the fluorine-29 nuclei to lose a proton, producing oxygen-28 nuclei.
But despite its number of protons and neutrons, the researchers discovered that oxygen-28 doesn’t seem to possess the stability that would be expected of doubly magic nuclei. Instead, the nuclei appear to form only fleetingly, before losing four neutrons to decay into oxygen-24. This suggests that the nuclei don’t actually have closed neutron shells and raises intriguing questions about the predicted energy gaps between the neutron shells.
To carry out the work, the researchers developed a state-of-the-art experimental setup for the direct detection of multiple neutrons. They note that this advance in multineutron-decay spectroscopy ‘opens up new perspectives in the investigation of other extremely neutron-rich systems’.
References
Y Kondo et al, Nature, 2023, DOI: 10.1038/s41586-023-06352-6
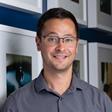
No comments yet