Long lived, high-energy excited states can give cobalt (III) complexes photocatalytic properties previously thought impossible, two US groups have shown. The findings could allow earth-abundant metal-based catalysts to replace precious metals for many important reactions and even to catalyse reactions not previously thought feasible.
Some complexes of precious metals such as ruthenium and iridium are extremely good photocatalysts because, when they absorb visible light, they enter long-lived ‘charge-transfer’ excited states that facilitate electron or energy transfer. But the electronic band structures of first-row transition metals like iron make such states unstable as they tend to decay into short-lived ‘ligand-field’ excited states. Efforts to use these metals in catalysis have largely focused on stabilising the charge-transfer states, but this often requires highly specialised ligands. ‘It becomes a trade-off: is it really a cheap alternative if you have to use really expensive ligands?’ notes Thomas Auvray of the University of Birmingham, UK.
Now, new findings from the groups of Michigan State University physical chemist Jim McCusker and Princeton University organic chemist David MacMillan could open the door to using earth-abundant metals as photocatalysts.
McCusker explains that during a project on the fundamental photophysics of transition metal complexes, he asked his student Atanu Ghosh to measure how the excited-state of an iron (II) complex – which has a lifetime of around one nanosecond – would be affected if the central iron atom was replaced by cobalt (III). ‘We knew the cobalt system had a higher-energy excited state, so I was guessing it would be a few hundred picoseconds,’ recalls McCusker. ‘[Ghosh] comes back and says it’s four nanoseconds. I said “That’s probably not right, so go back and re-measure it.”’
When the measurement was confirmed, however, the researchers worked out that the counter-intuitive finding could be explained by Marcus theory – a 1980s model that explains the rates of electron transfer reactions by linking the energy of excited states with their lifetimes. The theory predicts how, after decreasing with energy up to a certain point, the lifetime of an excited state can enter an ‘inverted’ region in which it increases once again. ‘The geometry of the excited state, once it’s relaxed, is very different from the geometry of the ground state,’ says McCusker. ‘In molecular systems, there tends to be a large barrier to doing that re-organisation.’
MacMillan, who won the 2021 chemistry Nobel prize for work on organocatalysis, recalls a social group call with McCusker and several friends during which he mentioned anomalous findings with cobalt catalysts in his own laboratory. ‘All of a sudden, the two ends connected,’ says MacMillan. ‘And they connected because Jim said: “Oh my God – this is what’s going on!”’
Using this understanding, the researchers developed cobalt-based substitutes for ruthenium and iridium complexes used in several industrially important reactions such as the arylation of ethers. They also demonstrated that, under blue light, a cobalt (III) complex with bipyridine ligands could catalyse the coupling of aryl amide to aryl boronic acid. This reaction had never previously been achieved and demonstrates the ability of their catalyst to build pharmaceutically important carbon–nitrogen bonds. ‘There are two steps to make this photocatalyst,’ says McCusker, ‘It’s a very garden-variety molecule – you can modify it very easily, which allows you to change the energy of the excited state, which means you can tune the excited state properties to the particular reaction that you might want to catalyse.’
The researchers hope this new paradigm for catalyst design will allow researchers to produce catalysts for other important reactions using other first-row transition metals. ‘Just imagine you’ve been playing chess for 20 years of your life, and all of a sudden someone comes along and says “Oh, by the way, we’ve introduced these three new rules that no-one ever knew about before,” and all of a sudden you can play the game in a completely different way … That’s the way the organic chemist looks at it,’ says MacMillan.
Auvray, who was not involved in the research, says that the findings open a path for ‘future design of earth-abundant photosensitisers for a wide range of transformations’.
’I’m not sure how straightforward it will be to convert to other metals,’ he says. ‘Cobalt (III) is a perfect case because the ligand field is so strong … There will be a need to look at other metals to see if they do present that inverted region. But I see very exciting possibilities.’
References
A Y Chan et al, Science, 2023, DOI: 10.1126/science.adj0612
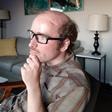
No comments yet